
- Chinese Optics Letters
- Vol. 23, Issue 2, 020602 (2025)
Abstract
Keywords
1. Introduction
Over the past decades, the development of fiber-optic networks has revolutionized the telecommunications industry, and optical fibers exist anywhere in modern society[1–4]. For the optical network, optical signals face the threat of being eavesdropped and attacked. How to secure optical networks has attracted extensive research, which can be categorized into two main areas: intrusion detection of optical fibers[5–7], and information safeguarding at the photonic layer[8–11]. Unfortunately, these two security measures operate independently and thus need separate deployment of intrusion detection systems and information safeguarding systems.
Enabling the secure optical transmission system to have the ability to detect attacks allows it to make dynamic adjustments to different types of attacks, improving the security, flexibility, and intelligence of the security transmission system. How to make a secure optical transmission system capable of detecting attacks is the same problem faced by fiber optic’s integrated communication and sensing.
A field trial demonstrated the feasibility of integrating distributed fiber optical sensing and high-speed communication in the same fiber, leveraging wavelength division multiplexing[12]. This pioneering effort shows that telecom fiber infrastructures can also provide sensing functions, opening up a new realm of possibilities for fiber-optic networks. Building upon this foundation, researchers have successfully utilized these fibers to measure and monitor seismic activities and water waves[13,14], showing the versatility and adaptability of fiber-optic sensing technologies. Additionally[15], it has demonstrated the simultaneous transmission of data and distributed vibration sensing within the same wavelength.
Sign up for Chinese Optics Letters TOC. Get the latest issue of Chinese Optics Letters delivered right to you!Sign up now
However, integrating sensing and communication within the same fiber offers significant advantages. However, this approach reveals the presence of a transmission link, potentially drawing the unwanted attention of an attacker. To mitigate this vulnerability, covert sensing and communication techniques present a possible solution.
In this Letter, an integrated covert sensing and communication method is proposed for the first time to the best of our knowledge. The integration of covert sensing and communication is presented in the same fiber, the same time, and the same wavelength. A proof-of-concept experiment is set up to verify the feasibility of the proposed method. The influence of the noise on the sensing, which is introduced by communication is investigated. In addition, the influence of the structure of the interferometer on communication performance is also studied.
2. Principle and System Model
The configuration of the optical covert sensing and communication system is shown in Fig. 1, which consists of a public communication channel, a covert communication channel, and a sensing channel based on a Sagnac interferometer[16].
Figure 1.Setup of the proof-of-concept experiment. ASE, amplified spontaneous emission; PPG, pulse pattern generator; SMF, single-mode fiber; EDFA, erbium-doped fiber amplifier; PD, photon detector; OF, optical filter; BER, bit error rate; DCF, dispersion compensation fiber; TX, transmitter; RX, receiver.
The public communication channel, designed for open transmission, comprises a public transmitter, a 25-km span of G.652 single-mode fiber (SMF), and a public receiver. In the transmitter of the covert communication channel, the amplified spontaneous emission (ASE) light without optical filtering is modulated by 1.25 Gbps signals from a pulse pattern generator (PPG) with on-off keying. Then, the modulated ASE light is spread by an SMF span in the time domain, and the spread ASE light is sent to the Sagnac structure through a
In the sensing receiver, the received signal is filtered by an optical filter (OF) to suppress the public optical signal and out-of-band optical noise. After the signal output from the OF, it is amplified by an erbium-doped fiber amplifier (EDFA) and sent into a photon detector (PD) for vibration sensing along the fiber. In the communication receiver, the received signal is compressed by a dispersion compensation fiber (DCF) in the time domain, and then the compressed signal is filtered by an OF to suppress the public signal and noise. The filtered signal is then amplified by the EDFA and sent to the PD for detection. The detected signal is then fed into a bit error rate (BER) tester for performance testing.
In the transmission link, the Sagnac interferometer is constructed jointly by the transmission fiber and the optical coupler to sense the vibration along the fiber. Therefore, the covert signals can perform both covert communication and covert sensing functions at the same time and with the same wavelength.
In the Sagnac interferometer, the output of the modulated ASE light is split into two parts and directed into two counter-propagating directions along the Sagnac loop. When the Sagnac interferometer is placed in a silent environment, the optical paths of these two counter-propagating parts are identical, so when they are recombined and interfere with the sensing output, the output intensity does not change. In this experiment, a 1-meter-long fiber optic cable of the Sagnac loop is wound around a piezoelectric transducer (PZT) ring with a 130 kHz resonance frequency, which is used to generate the vibration signal and stretch the fiber, thus changing the phase of the transmitted light. When selecting a PZT, it is necessary to consider that its resonance frequency is as large as possible to avoid drowning the components of the null frequency. Due to the difference in the time taken by the clockwise and counterclockwise propagating lights to travel from the coupler to the location where vibration occurs, the phase changes experienced by the two are also different, resulting in a phase difference.
Assuming the region affected by the vibration is much smaller than the overall interferometer length, the phase difference can be written as
In the case that the vibration signal is
This part is oscillating with an amplitude of
3. Results and Analysis
After setting up the proof-of-concept experiment, our investigation focused on several key aspects. First, we examined the feasibility of utilizing the modulated ASE signal for covert optical sensing purposes. Second, we explored the impact of electrical noise, introduced through the modulation process, on the accuracy of optical sensing. Additionally, we assessed the performance of covert sensing under power constraints.
This analysis was divided into two main sections. The first section investigated the impact of communication on covert sensing, while the second section examined the impact of vibrations on the transmission performance.
3.1. The impact of communication on covert sensing
Here, a 1 m-long fiber optic cable of the Sagnac loop is wound around a PZT ring with a 130 kHz resonance frequency. The PZT is driven by a series of electrical pulses with a frequency of 100 Hz, a duty cycle of 0.01%, and an amplitude of 2 V. This frequency is chosen because it allows each electrical pulse applied to the PZT sufficient time to decay and require a low storage depth on the oscilloscope. Here, the initial values of
In the sensing receiver, the detected waveform in the time domain and the corresponding power spectrum density are shown in Fig. 2. As can be seen, the vibration signal with a frequency of 100 Hz is clear from the time domain waveform in Fig. 2(a). To reduce the variance of the frequency estimate and eliminate the effects of noise, the Welch method is used to produce the frequency spectrum. The spectrum in Fig. 2(b) reveals a prominent series of null frequency points, and the vibration location can thus be accurately calculated through Eq. (5). Therefore, the covert signal can be used for sensing the vibration in the transmission link.
Figure 2.Waveform and frequency of the signal.
Communication noise is introduced by modulation and its source is the modulating signal. Since the frequency of the modulating signal is relatively high compared to the sensing signal, the additional noise introduced by the modulation interferes less with the low-frequency sensing signal. Noise is filtered out during sensing analysis because of its high frequency. Environmental factors can also affect sensing, such as temperature, but temperature changes slowly and therefore has less impact on the measurement of the sensing signal.
To further ascertain the viability of covert sensing, the fiber length was adjusted by changing the length of the short SMF span from 0 to 5 km. The results are depicted in Fig. 3. As can be seen from Fig. 3(a), a linear increase in the null frequency is observed with the progression of
Figure 3.Experimental results of different positions. (a) The measured null frequency and (b) the error of the calculated position.
In addition, comparing the sensing measurement with and without the modulated signal from the PPG, the null frequency in spectrum and measurement error show a tiny difference between them when
3.2. The impact of sensing on covert communication
To verify the feasibility of covert communication when integrated with covert sensing mechanisms, here we analyze the impact of sensing on covert communication. From the structure of the Sagnac interferometer, no anti-clock signal enters the covert channel receiver. Consequently, the signal received at the covert channel receiver in this proposed scheme remains the same as that in a traditional unidirectional transmission system.
From the perspective of vibration analysis, the frequency of the vibration signal is maintained at 100 Hz. Figure 4 illustrates the BER curves for the covert channel with and without vibration. It is evident from the figure that the two BER curves are nearly superimposable, indicating that the vibration exerts minimal influence on the transmission performance of the covert channel. Because the ASE carrier is integrated for sensing and communication, sensing does not have an additional impact on communication compared to other sensing methods such as optical time-domain reflectometers.
Figure 4.BER curves of the covert channel with and without vibration.
In addition, the BER curves of the public channel under vibration are measured and shown in Fig. 5. The average optical power of a public channel is
Figure 5.BER curves of the public channel under vibration.
4. Conclusions
In this Letter, an integrated optical covert sensing and communication method is proposed and demonstrated by a proof-of-concept experiment. The sensing and communication systems have the same optical source based on the ASE light, and the optical covert sensor is based on a Sagnac structure. The covert communication signal has less power than the public channel. As the experimental results show, covert sensing has little impact on covert communication, and covert communication is the same when the order of null frequency is larger than 1. The integrated optical covert sensing and communication systems are working at the same time and the same wavelength. This work may simplify the system structure to enrich the system functions of optical communications.
References
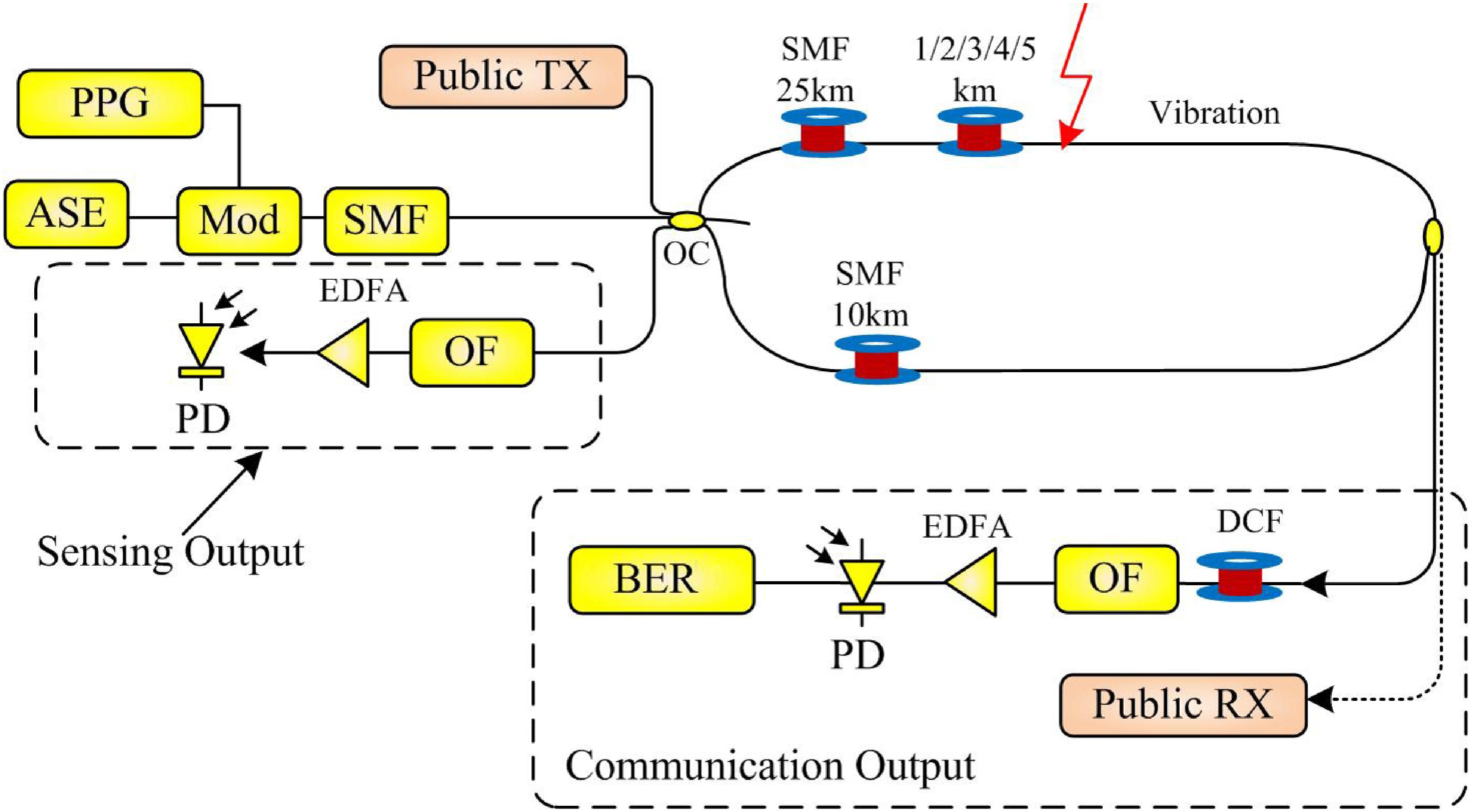
Set citation alerts for the article
Please enter your email address