Junho Ahn, Minseong Kim, Chulhong Kim, Wonseok Choi, "In vivo multi-scale clinical photoacoustic imaging for analysis of skin vasculature and pigmentation: a comparative review," Adv. Imaging 1, 032002 (2024)

Search by keywords or author
- Advanced Imaging
- Vol. 1, Issue 3, 032002 (2024)
![PAI modalities in skin imaging. (a) A schematic of the PAI principle (left) and the optical absorption spectra of HbO2, HbR, and melanin (right). The spectra data are from http://omlc.ogi.edu. (b) Conceptual schematics and example images of the vessel and melanin in the skin for each PAI modality. US, ultrasound; PA, photoacoustic; PAI, photoacoustic imaging; PAM, photoacoustic microscopy; PAMes, photoacoustic mesoscopy; PAT, photoacoustic tomography; HbR, deoxy-hemoglobin; HbO2, oxy-hemoglobin; TR, transducer; EP, epidermis; DR, dermis; ST, subcutaneous tissue; FOV, field of view. The figures were reproduced with permission from Refs. [100] (Copyright © 2021 Optical Society of America), [120] (Copyright © 2021 Optical Society of America), [112] (Copyright © 2022 by the authors. Licensee MDPI, Basel, Switzerland), and [122] (Copyright © by the authors).](/richHtml/ai/2024/1/3/032002/img_001.png)
Fig. 1. PAI modalities in skin imaging. (a) A schematic of the PAI principle (left) and the optical absorption spectra of , HbR, and melanin (right). The spectra data are from http://omlc.ogi.edu . (b) Conceptual schematics and example images of the vessel and melanin in the skin for each PAI modality. US, ultrasound; PA, photoacoustic; PAI, photoacoustic imaging; PAM, photoacoustic microscopy; PAMes, photoacoustic mesoscopy; PAT, photoacoustic tomography; HbR, deoxy-hemoglobin; , oxy-hemoglobin; TR, transducer; EP, epidermis; DR, dermis; ST, subcutaneous tissue; FOV, field of view. The figures were reproduced with permission from Refs. [100] (Copyright © 2021 Optical Society of America), [120] (Copyright © 2021 Optical Society of America), [112] (Copyright © 2022 by the authors. Licensee MDPI, Basel, Switzerland), and [122] (Copyright © by the authors).
![PAM techniques for improved visualization of skin structures. (a) An OR-PAM system integrated with a photoplethysmography (PPG) sensor. Simultaneous real-time monitoring of PA and PPG signals from superficial dermal vessels in fingers accurately estimated the heartbeat rate (∼1.35 Hz) of healthy volunteers. (b) Imaging probe configuration and example images of a healthy volunteer’s palm skin using the dual-modal PA/US dermoscope. The transducer consisted of a PVDF transducer for PA/US signal reception and a PZT transducer for US signal transmission. PA and US images were able to depict shallow microvasculature and deeper skin structures in the palm, respectively. M, mirror; FC, fiber coupler/collimator; P, prism; GS, galvanometer scanner; WT, water tank; LS, linear stage; RUT, ring-shaped US transducer; OW, optical window; PBM, parabolic mirror; PVDF, polyvinylidene fluoride; PZT, lead zirconate titanate; PA, photoacoustic; US, ultrasound; SC, stratum corneum. The figures were reproduced with permission from Refs. [96] (Copyright © 2022 by the authors. Published by Elsevier GmbH) and [97] (Copyright © 2020 WILEY-VCH Verlag GmbH & Co. KGaA, Weinheim).](/richHtml/ai/2024/1/3/032002/img_002.png)
Fig. 2. PAM techniques for improved visualization of skin structures. (a) An OR-PAM system integrated with a photoplethysmography (PPG) sensor. Simultaneous real-time monitoring of PA and PPG signals from superficial dermal vessels in fingers accurately estimated the heartbeat rate ( ) of healthy volunteers. (b) Imaging probe configuration and example images of a healthy volunteer’s palm skin using the dual-modal PA/US dermoscope. The transducer consisted of a PVDF transducer for PA/US signal reception and a PZT transducer for US signal transmission. PA and US images were able to depict shallow microvasculature and deeper skin structures in the palm, respectively. M, mirror; FC, fiber coupler/collimator; P, prism; GS, galvanometer scanner; WT, water tank; LS, linear stage; RUT, ring-shaped US transducer; OW, optical window; PBM, parabolic mirror; PVDF, polyvinylidene fluoride; PZT, lead zirconate titanate; PA, photoacoustic; US, ultrasound; SC, stratum corneum. The figures were reproduced with permission from Refs. [96] (Copyright © 2022 by the authors. Published by Elsevier GmbH) and [97] (Copyright © 2020 WILEY-VCH Verlag GmbH & Co. KGaA, Weinheim).
![Photoacoustic microscopic biopsy (PAMB) technique for port wine stain (PWS) lesion imaging. Compared to normal skins, PWS skins have dilated vascular structure in the dermal layer. MAP, maximum amplitude projection; EP, epidermis; DR, dermis; SB, stratum basale; SC, stratum corneum. The figures were reproduced with permission from Ref. [100] (Copyright © 2021 Optical Society of America).](/Images/icon/loading.gif)
Fig. 3. Photoacoustic microscopic biopsy (PAMB) technique for port wine stain (PWS) lesion imaging. Compared to normal skins, PWS skins have dilated vascular structure in the dermal layer. MAP, maximum amplitude projection; EP, epidermis; DR, dermis; SB, stratum basale; SC, stratum corneum. The figures were reproduced with permission from Ref. [100] (Copyright © 2021 Optical Society of America).
![(a) Cross-sectional RSOM image composed of two bandwidth signal images and a binary image of the high-frequency signal within ROI. The combined image of high-frequency signal (green, representing small vessels) and low-frequency signal (red, representing large vessels) shows the boundary between epidermis and dermis. The binary image is used to calculate the vessel fragmentation value. (b) The process of skin biomarker quantification in cross-sectional RSOM images. First, the EP and DR regions are divided, and the EP thickness and the EP signal density are calculated. Then, the vessel numbers are calculated by counting the vessel branches (red dots) in the DR area, and the total blood volume is calculated from the binary image. RSOM, raster scan optoacoustic mesoscopy; ROI, region of interest; EP, epidermis; DR, dermis; VS, vessel segmentation; BC, biomarker computation. The figures were reproduced with permission from Refs. [101] (Copyright © 2020 by the authors. Contact Dermatitis published by John Wiley & Sons Ltd.) and [107] [Copyright © 2023 by the author(s)].](/Images/icon/loading.gif)
Fig. 4. (a) Cross-sectional RSOM image composed of two bandwidth signal images and a binary image of the high-frequency signal within ROI. The combined image of high-frequency signal (green, representing small vessels) and low-frequency signal (red, representing large vessels) shows the boundary between epidermis and dermis. The binary image is used to calculate the vessel fragmentation value. (b) The process of skin biomarker quantification in cross-sectional RSOM images. First, the EP and DR regions are divided, and the EP thickness and the EP signal density are calculated. Then, the vessel numbers are calculated by counting the vessel branches (red dots) in the DR area, and the total blood volume is calculated from the binary image. RSOM, raster scan optoacoustic mesoscopy; ROI, region of interest; EP, epidermis; DR, dermis; VS, vessel segmentation; BC, biomarker computation. The figures were reproduced with permission from Refs. [101] (Copyright © 2020 by the authors. Contact Dermatitis published by John Wiley & Sons Ltd.) and [107] [Copyright © 2023 by the author(s)].
![PAT techniques for clinical skin vasculature imaging. (a) MSOT principle and image processing step. (b) MSOT images of HbR, HbO2, and HbT for healthy volunteer, and patients with stable SSc and progressive SSc. (c1) MSOT images of Sp and Dp. (c2) MSOT images of HbO2 and HbR during the reactive hyperemia. PAT, photoacoustic tomography; US, ultrasound; MSOT, multi-spectral optoacoustic tomography; MSP, multispectral pulsed; HbR, deoxy-hemoglobin; HbO2, oxy-hemoglobin; HbT, total hemoglobin; SSc, systemic sclerosis; Sp, superficial plexus; Dp, deep plexus. The figures were reproduced with permission from Refs. [109] (Copyright © 2018 WILEY-VCH Verlag GmbH & Co. KGaA, Weinheim) and [112] (Copyright © 2022 by the authors; Licensee MDPI, Basel, Switzerland).](/Images/icon/loading.gif)
Fig. 5. PAT techniques for clinical skin vasculature imaging. (a) MSOT principle and image processing step. (b) MSOT images of HbR, , and HbT for healthy volunteer, and patients with stable SSc and progressive SSc. (c1) MSOT images of Sp and Dp. (c2) MSOT images of and HbR during the reactive hyperemia. PAT, photoacoustic tomography; US, ultrasound; MSOT, multi-spectral optoacoustic tomography; MSP, multispectral pulsed; HbR, deoxy-hemoglobin; , oxy-hemoglobin; HbT, total hemoglobin; SSc, systemic sclerosis; Sp, superficial plexus; Dp, deep plexus. The figures were reproduced with permission from Refs. [109] (Copyright © 2018 WILEY-VCH Verlag GmbH & Co. KGaA, Weinheim) and [112] (Copyright © 2022 by the authors; Licensee MDPI, Basel, Switzerland).
![Switchable OR-AR PAM techniques for skin vasculature and pigment imaging. (a) Switchable OR-AR PAM technique that alters the focusing mode by axially translating the depth of the fixed focus. (b1)–(b3) Autofocusing PAM technique using a voltage-controlled liquid lens. (b1) The focal sizes of the optical and acoustic beams were controlled by the input voltage at the electrodes. (b2) Phantom imaging demonstrated that autofocusing improved the imaging depth compared to the fixed focus mode. (b3) A healthy volunteer’s palm skin was imaged in different layers, where SC/SB layers and Va layers contained the melanin and vasculature, respectively. OR, optical resolution; AR, acoustic resolution; EP, epidermis; DP, dermal papillae; RD, reticular dermis; Hy, hypodermis; SC, stratum corneum; SB, stratum basale; SVP, superficial vascular plexus; DVP, deep vascular plexus; E-D, epidermis-dermis junction; VN, vascular network; PVDF, polyvinylidene fluoride. The figures were reproduced with permission from Refs. [114] [Copyright © 2020 by the author(s)] and [115] (Copyright © 2022 Wiley-VCH GmbH).](/Images/icon/loading.gif)
Fig. 6. Switchable OR-AR PAM techniques for skin vasculature and pigment imaging. (a) Switchable OR-AR PAM technique that alters the focusing mode by axially translating the depth of the fixed focus. (b1)–(b3) Autofocusing PAM technique using a voltage-controlled liquid lens. (b1) The focal sizes of the optical and acoustic beams were controlled by the input voltage at the electrodes. (b2) Phantom imaging demonstrated that autofocusing improved the imaging depth compared to the fixed focus mode. (b3) A healthy volunteer’s palm skin was imaged in different layers, where SC/SB layers and Va layers contained the melanin and vasculature, respectively. OR, optical resolution; AR, acoustic resolution; EP, epidermis; DP, dermal papillae; RD, reticular dermis; Hy, hypodermis; SC, stratum corneum; SB, stratum basale; SVP, superficial vascular plexus; DVP, deep vascular plexus; E-D, epidermis-dermis junction; VN, vascular network; PVDF, polyvinylidene fluoride. The figures were reproduced with permission from Refs. [114] [Copyright © 2020 by the author(s)] and [115] (Copyright © 2022 Wiley-VCH GmbH).
![Bifocal dual-wavelength PAM technique for port wine stain (PWS) characterization. (a) Two optical wavelengths, 532 and 1064 nm, were focused at different depths and superposed to provide elongated focal zone and deep imaging depth up to 3 mm. The epidermis (EP) and superficial vascular plexus (SVP) were imaged at 532 nm and deep vascular plexus (DVP) at 1064 nm. (b) Compared to normal skin, PWS skin expressed less melanin in the EP layer and more vasculature in the SVP and DVP layers. DE, dermis; Hy, hypodermis; SC, stratum corneum; ED, epidermal-dermal junction; PWS, port wine stain; PA, photoacoustic; and a.u., arbitrary unit. The figures were reproduced with permission from Ref. [118] (Copyright © 2021 European Academy of Dermatology and Venereology).](/Images/icon/loading.gif)
Fig. 7. Bifocal dual-wavelength PAM technique for port wine stain (PWS) characterization. (a) Two optical wavelengths, 532 and 1064 nm, were focused at different depths and superposed to provide elongated focal zone and deep imaging depth up to 3 mm. The epidermis (EP) and superficial vascular plexus (SVP) were imaged at 532 nm and deep vascular plexus (DVP) at 1064 nm. (b) Compared to normal skin, PWS skin expressed less melanin in the EP layer and more vasculature in the SVP and DVP layers. DE, dermis; Hy, hypodermis; SC, stratum corneum; ED, epidermal-dermal junction; PWS, port wine stain; PA, photoacoustic; and a.u., arbitrary unit. The figures were reproduced with permission from Ref. [118] (Copyright © 2021 European Academy of Dermatology and Venereology).
![Classification of melasma lesion types using PAM. (a) The PAM images are from a patient with epidermal M + V type melasma, showing denser melanin in the epidermal layer (z=0–165 μm) and denser and thicker vessels in the dermal layer (z=165–1050 μm). (b) All types of melasma lesions showed a significant increase in the PA amplitude of melanin. The vascular diameter and density were not significantly changed in the epidermal M and mixed M types, but the M + V type had a significant increase in the two vascular metrics. MAP, maximum amplitude projection; a.u., arbitrary unit; and PA, photoacoustic. The figures were reproduced with permission from Ref. [119] (Copyright © 2023 by the authors).](/Images/icon/loading.gif)
Fig. 8. Classification of melasma lesion types using PAM. (a) The PAM images are from a patient with epidermal M + V type melasma, showing denser melanin in the epidermal layer ( ) and denser and thicker vessels in the dermal layer ( ). (b) All types of melasma lesions showed a significant increase in the PA amplitude of melanin. The vascular diameter and density were not significantly changed in the epidermal M and mixed M types, but the M + V type had a significant increase in the two vascular metrics. MAP, maximum amplitude projection; a.u., arbitrary unit; and PA, photoacoustic. The figures were reproduced with permission from Ref. [119] (Copyright © 2023 by the authors).
![RSOM images of a melanoma lesion and the surrounding skin tissue. (a) A photograph of a melanoma lesion. Arrows indicate the three scanning positions. The red rectangle depicts the FOV of 4 mm×2 mm. (b) Histological images of the melanoma sample corresponding to the area with label 1 in (a). (c)–(e) Cross-sectional RSOM images of the three scanned regions [labels 1, 2, and 3 in (a)]. (f)–(h) Maximum amplitude projection (MAP) images corresponding to the epidermal (EP) layer of (c)–(e). (i)–(k) MAP images corresponding to the dermal (DR) layer of (c)–(e). The white dashed lines in (g) indicate the boundary between the pigmented lesion and the surrounding skin tissue. Comparing the dermal vascular structures of (i)–(k), a dense dotted vascular pattern is clearly visible in the tumor base area. The figures were reproduced with permission from Ref. [120] [Copyright © 2022 by the author(s)].](/Images/icon/loading.gif)
Fig. 9. RSOM images of a melanoma lesion and the surrounding skin tissue. (a) A photograph of a melanoma lesion. Arrows indicate the three scanning positions. The red rectangle depicts the FOV of . (b) Histological images of the melanoma sample corresponding to the area with label 1 in (a). (c)–(e) Cross-sectional RSOM images of the three scanned regions [labels 1, 2, and 3 in (a)]. (f)–(h) Maximum amplitude projection (MAP) images corresponding to the epidermal (EP) layer of (c)–(e). (i)–(k) MAP images corresponding to the dermal (DR) layer of (c)–(e). The white dashed lines in (g) indicate the boundary between the pigmented lesion and the surrounding skin tissue. Comparing the dermal vascular structures of (i)–(k), a dense dotted vascular pattern is clearly visible in the tumor base area. The figures were reproduced with permission from Ref. [120] [Copyright © 2022 by the author(s)].
![UWSB-RSOM system configuration and cross-sectional USWB-RSOM images over 5 wavelengths. (a) Schematic of UWSB-RSOM and a structure of the imaging target (skin). (b1)–(b6) Cross-sectional RSOM images of skin over 5 wavelengths. (b1), (b2) Distribution of hemoglobin and melanin in the skin at 515 and 532 nm. Arrows indicate the position of hair. (b3) Melanin in the epidermis and hair at 650 nm. (b4) Subcutaneous fat, sebaceous glands (marked with arrows 1 and 2), and water content in the epidermis at 1210 nm; arrow 3, sebum content on the hair shaft. (b5) Water distributed in the epidermis at 1450 nm. (b6) Composite of images at all five wavelengths. ADC, analog-to-digital converter; AMP, 60 dBm amplifier; CL, collimating lens; D, dermis; EP, epidermis; FB, fiber bundle; FL, focusing lens; H, UWSB-RSOM holder; HPF, high-pass filter; HS, hair shaft; HB, hair bulb; IU, detachable interface unit; M, mirror; PBS, polarizing beam splitter; S, schematic of human skin; SF, subcutaneous fat; SG, sebaceous glands; UT, ultrasound transducer. The figures were reproduced with permission from Ref. [121] (Copyright © 2019 Optical Society of America).](/Images/icon/loading.gif)
Fig. 10. UWSB-RSOM system configuration and cross-sectional USWB-RSOM images over 5 wavelengths. (a) Schematic of UWSB-RSOM and a structure of the imaging target (skin). (b1)–(b6) Cross-sectional RSOM images of skin over 5 wavelengths. (b1), (b2) Distribution of hemoglobin and melanin in the skin at 515 and 532 nm. Arrows indicate the position of hair. (b3) Melanin in the epidermis and hair at 650 nm. (b4) Subcutaneous fat, sebaceous glands (marked with arrows 1 and 2), and water content in the epidermis at 1210 nm; arrow 3, sebum content on the hair shaft. (b5) Water distributed in the epidermis at 1450 nm. (b6) Composite of images at all five wavelengths. ADC, analog-to-digital converter; AMP, 60 dBm amplifier; CL, collimating lens; D, dermis; EP, epidermis; FB, fiber bundle; FL, focusing lens; H, UWSB-RSOM holder; HPF, high-pass filter; HS, hair shaft; HB, hair bulb; IU, detachable interface unit; M, mirror; PBS, polarizing beam splitter; S, schematic of human skin; SF, subcutaneous fat; SG, sebaceous glands; UT, ultrasound transducer. The figures were reproduced with permission from Ref. [121] (Copyright © 2019 Optical Society of America).
![PAT techniques for melanoma imaging. (a) Clinical photograph of a melanoma. (b) 3D PA scan image of the black dashed region in (a). (c) Co-registration of PA and B-mode US images of in situ melanoma on the upper left extremity. (d) Clinical photograph of an in situ type of melanoma. (e) PAampMAP and (f) PAunmixedMAP images of the red dashed region in (d). (g) PAamp/US overlaid and (h) PAunmixed/US overlaid B-scan images from the white dashed lines in (e) and (f). PA, photoacoustic; US, ultrasound; PAamp, PA amplitude; PAunmixed, PA unmixed melanoma; MAP, maximum amplitude projection. The figures were reproduced with permission from Refs. [122] (Copyright © by the authors) and [125] (Copyright © 2020 European Academy of Dermatology and Venereology).](/Images/icon/loading.gif)
Fig. 11. PAT techniques for melanoma imaging. (a) Clinical photograph of a melanoma. (b) 3D PA scan image of the black dashed region in (a). (c) Co-registration of PA and B-mode US images of in situ melanoma on the upper left extremity. (d) Clinical photograph of an in situ type of melanoma. (e) PAampMAP and (f) PAunmixedMAP images of the red dashed region in (d). (g) PAamp/US overlaid and (h) PAunmixed/US overlaid B-scan images from the white dashed lines in (e) and (f). PA, photoacoustic; US, ultrasound; PAamp, PA amplitude; PAunmixed, PA unmixed melanoma; MAP, maximum amplitude projection. The figures were reproduced with permission from Refs. [122] (Copyright © by the authors) and [125] (Copyright © 2020 European Academy of Dermatology and Venereology).
![PAT techniques for skin lesions. (a) Comparison of combined in vivo US/PA measurement on human nevi (left) and the corresponding histological measurements (right). (b) PA imaging of nevus with atypical proliferation (left) and basal cell carcinoma (right). The nevus presents chaotic and prominent blood vessels outside the melanin area (yellow), HbO2 (red), and Hb (blue) blood vessels with enlarged diameter. The carcinoma shows abundant HbO2 signal (red) inside the melanin area, demonstrating a converging network of melanin (yellow), HbO2 (red), and HbR (blue). PAT, photoacoustic tomography; US, ultrasound; PA, photoacoustic; HbO2, oxy-hemoglobin; HbR, deoxy-hemoglobin. The figures were reproduced with permission from Refs. [126] [Copyright © 2023 by the author(s)] and [131] (Copyright © 2022 Acta Dermato-Venereologica).](/Images/icon/loading.gif)
Fig. 12. PAT techniques for skin lesions. (a) Comparison of combined in vivo US/PA measurement on human nevi (left) and the corresponding histological measurements (right). (b) PA imaging of nevus with atypical proliferation (left) and basal cell carcinoma (right). The nevus presents chaotic and prominent blood vessels outside the melanin area (yellow), (red), and Hb (blue) blood vessels with enlarged diameter. The carcinoma shows abundant signal (red) inside the melanin area, demonstrating a converging network of melanin (yellow), (red), and HbR (blue). PAT, photoacoustic tomography; US, ultrasound; PA, photoacoustic; , oxy-hemoglobin; HbR, deoxy-hemoglobin. The figures were reproduced with permission from Refs. [126] [Copyright © 2023 by the author(s)] and [131] (Copyright © 2022 Acta Dermato-Venereologica).
![Specification plots relating the spatial resolution, imaging depth, and lateral FOV of clinical PA skin imaging systems. The overall relationship of the imaging depth versus spatial resolution (left) and the lateral FOV versus spatial resolution (right) is presented. Dotted lines show reference tradeoff lines with a given ratio, and the blue dotted line is obtained from least-square fitting including both axial (triangle marker) and lateral (circle marker) resolutions. PAM, photoacoustic microscopy; PAMes, photoacoustic mesoscopy; PAT, photoacoustic tomography; FOV, field of view; PA, photoacoustic. (Refs. PAM- [95,97,99,100,114–119" target="_self" style="display: inline;">–119]; PAMes - [101,102,104,107,108,120,121]; PAT - [110,113,125–127" target="_self" style="display: inline;">–127,129].](/Images/icon/loading.gif)
Fig. 13. Specification plots relating the spatial resolution, imaging depth, and lateral FOV of clinical PA skin imaging systems. The overall relationship of the imaging depth versus spatial resolution (left) and the lateral FOV versus spatial resolution (right) is presented. Dotted lines show reference tradeoff lines with a given ratio, and the blue dotted line is obtained from least-square fitting including both axial (triangle marker) and lateral (circle marker) resolutions. PAM, photoacoustic microscopy; PAMes, photoacoustic mesoscopy; PAT, photoacoustic tomography; FOV, field of view; PA, photoacoustic. (Refs. PAM- [95,97,99,100,114– 119" target="_self" style="display: inline;">– 119 ]; PAMes - [101,102,104,107,108,120,121]; PAT - [110,113,125– 127" target="_self" style="display: inline;">– 127 ,129].
|
Table 1. Specifications of PAM Systems for Clinical Skin Imaging.
|
Table 2. Specifications of PAMes Systems for Clinical Skin Imaging.
|
Table 3. Specifications of PAT Systems for Clinical Skin Imaging.
|
Table 4. PAI Analysis Features of the Skin Vasculature.
|
Table 5. PAI Analysis Features of the Skin Vasculature and Pigmentation.
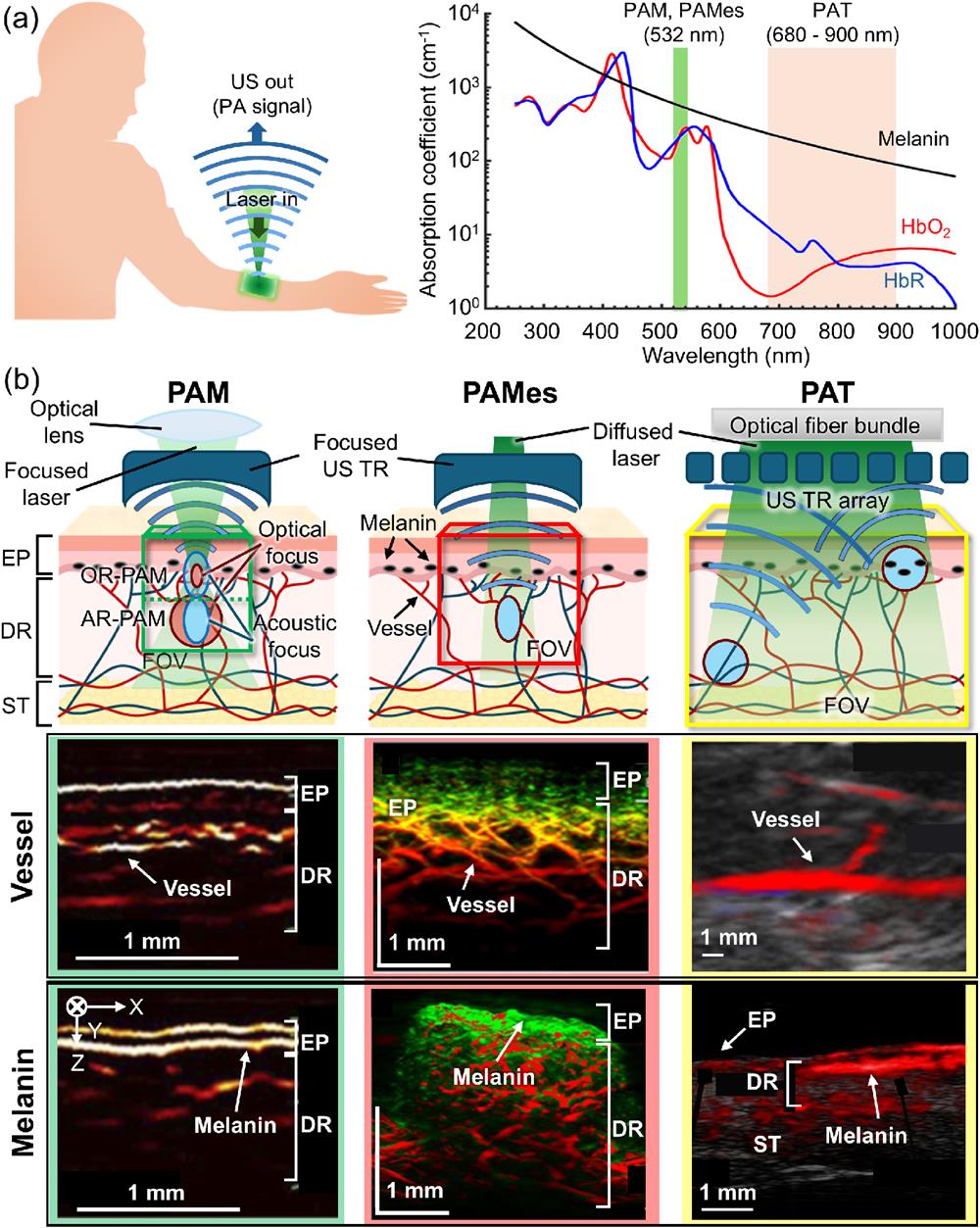
Set citation alerts for the article
Please enter your email address