Tong ZHANG, Qiaoya SHI, Ye FENG, Chen WANG, Yishan WANG, Caiwen MA. Fully Integrated Kerr-lens Mode-locked Ceramic Laser for Low-noise Clock (Invited)[J]. Acta Photonica Sinica, 2025, 54(2): 0254103

Search by keywords or author
- Acta Photonica Sinica
- Vol. 54, Issue 2, 0254103 (2025)
Note: This section is automatically generated by AI . The website and platform operators shall not be liable for any commercial or legal consequences arising from your use of AI generated content on this website. Please be aware of this.
Abstract
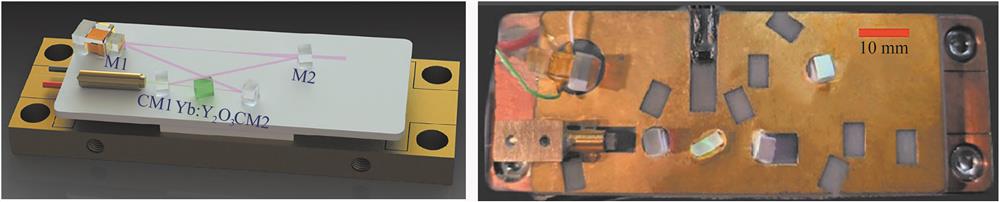
Set citation alerts for the article
Please enter your email address