
- Acta Photonica Sinica
- Vol. 50, Issue 3, 62 (2021)
Abstract
Keywords
0 Introduction
Due to numerous advantages, such as compact structure, low cost, high efficiency, high pulse peak power, high pulse repetition, and good beam quality, Diode-Pumped Solid-State (DPSS) passively Q-switched lasers have been widely used in industrial, military, medical, and scientific research fields[
The pulsed lasers described above generally refer to pulsed lasers having a stable pulse amplitude, which are called stable pulsed lasers, and there have been many studies on stable pulsed lasers. In fact, there have been another type of pulsed lasers, Pulse Amplitude Chaos (PAC) lasers [
In this work, by adopting a laser diode emitting around 795 nm as a pump source, a Nd:LMA disordered crystal as a laser gain medium, a SESAM as a saturable absorber, we have experimentally demonstrated a Nd:LMA pulsed laser. Experimental results indicate that the diode-pumped Nd:LMA laser can operate at Q-switched state or PAC state depended on the pump power, where the chaos of PAC laser is judged through analyzing the autocorrelation curve, phase portrait, power spectrum and stochastic histogram of the Pulse-Peak Time Series (PPTS). For the Q-switched state and PAC state, the ranges of pump power are 4.8~8.6 W and 8.7~10.5 W, respectively. The maximum average output power of PAC laser arrives at about 814 mW under a pump power of 10.5 W.
1 Experimental setup
A uncoated optically polished a-cut Nd:LMA disordered crystal with dimensions of 3 mm×3 mm×5 mm and Nd3+ doping concentration of 5 at.% is used as a laser gain medium in the experimental system. The σ polarized emission and absorption spectra of the Nd:LMA disordered crystal have been measured, which includes two emission peaks at about 1 055 nm and 1 082 nm in the range of 1 000~1 100 nm and one absorption peak around 795 nm [
The experimental setups for the diode-pumped Nd:LMA laser operating at CW state and pulsed states, including Q-switched state and PAC state, are illustrated in Fig. 1. Under the CW state shown as Fig.1(a), a three-mirror-folded cavity is utilized, which is composed of a pump mirror 1(M1), a concave mirror 2(M2), and an output coupler (OC). M1 is a plane dichroic mirror, possessing high transmission at 796 ± 10 nm and high reflection at 1 000~1 100 nm. M2 has a Radius of Curvature (ROC) of 200 mm and a high reflection coating at 940~1 100 nm. OC is a plane mirror with the transmission of 15% at 1 000~1 100 nm. Under the pulsed states shown as Fig.1 (b), the laser cavity is composed of a pump mirror (M1), two concave mirrors (M2, M3), an output coupler (OC), and a SESAM. M3 has a high reflection coating at 900~1 100 nm and a ROC of 300 mm. The SESAM (BATOP GmbH) operates at 1 030~1 100 nm and has a modulation depth of 0.4%, an absorbance of 0.7%, a saturation fluence of 130 µJ/cm2, and a relaxation time of 1 ps. In the experiment, the output characteristics of Nd:LMA laser are analyzed in the optical domain and time domain by employing an optical spectrum analyzer (Ando AQ6317C, 600~1 750 nm) and an oscilloscope (Agilent Technologies DS09254A), respectively. Laser powers are measured by using an optical power meter (Thorlabs-PM100D, Thorlabs-CAL).

2 Experimental results and discussion
At first, the CW Nd:LMA laser performances are investigated by using the experimental setup without introducing SESAM (shown as Fig.1(a)). Fig.2(a) displays the dependences of the average output power on the pump power. Although the maximum output power of the laser diode can reach 12.0 W, in order to avoid damage the Nd:LMA disordered crystal, the pump power does not exceed 10.5 W during the experiment. From Fig.2(a), one can see that the pump threshold power is about 0.8 W and once the pump power exceeds 0.8 W, the average output power increases almost linearly with the increase of the pump power. When the pump power is increased to 10.5 W, the average output power arrives at about 1.0 W. And the corresponding slope efficiency and light-to-light efficiency are calculated to be about 10.7% and 9.5%, respectively. Fig.2(b) shows the measured optical spectrum for the CW Nd:LMA laser under a pump power of 6.0 W. The center wavelength of the CW Nd:LMA laser is about 1 056 nm.

In order to make the Nd:LMA laser operates at pulsed states, a concave mirror (M3) and a SESAM are introduced to the laser cavity shown as Fig.1 (b). With this experimental system, the Nd:LMA laser can operate at CW state, Q-switched state or PAC state, which is depended on the pump power. Fig.3 measures the average output power as a function of the pump power for the Nd:LMA laser operating at the three states and also shows the pump power ranges for the three states. Due to the M3 and SESAM introducing more intracavity loss, the pump threshold power increases up to about 1.8 W. As the pump power increases, the average output power increases in volatility. When the pump power is more than the pump threshold power, but relatively low (in the range of 1.8~4.7 W), the Nd:LMA laser operates at a CW state . And with the increase of the pump power (in the range of 4.8~8.6 W), the laser can enter a Q-switched state . When the pump power is in the range of 8.7~10.5 W, the Nd:LMA laser enters in a PAC state . The maximum average output power of the Nd:LMA laser operating at a Q-switched state and a PAC state are about 613 mW under a pump power of 8.6 W and 814 mW under a pump power of 10.5 W, respectively.

In order to analyze the performances of the Nd:LMA laser operating at a Q-switched state, the pulse time series, optical spectrum, and beam profile are measured and recorded under a pump power of 5.1 W. Fig.4(a) and 4(b) show the pulse time series with 0.001 s and 0.000 1 s time scales, respectively. From these two diagrams, one can see that the Nd:LMA Q-switched laser remains relatively stable and there are no multiple pulses, no harmonics, and no pulse splitting. Under this condition, the measured repetition frequency and pulse width are 117.7 kHz and 3.5 µs, respectively. Fig.4(c) shows the optical spectrum with a center wavelength at about 1 056 nm. The spatial distribution of the beam measured by a CCD camera shown as Fig.4(d), which indicates that the laser operates at a fundamental mode. Generally, for Q-switched lasers, the dependences of the repetition frequency and pulse width with the pump power is also an important parameter. Hence, we also record the repetition frequencies and pulse widths under different pump powers shown as Fig.5. The pulse widths gradually decrease while the repetition frequencies gradually increase with the increase of the pump power, which follows the laws of Q-switching. The variation ranges of the pulse widths and repetition frequencies are about 4.0~2.2 µs and 114.6~157.1 kHz, respectively.


When the pump power is in the range of 8.7~10.5 W, the pulse amplitudes of the Nd:LMA laser present as irregular and random distribution. In order to analyze this special performances of the Nd:LMA laser, the pulse time series and optical spectrum, and beam profile are measured and recorded under a pump power of 10.2 W. Fig.6(a), 6(b) and 6(c) represent the pulse time series with 0.05 s, 0.000 2 s, and 0.000 02 s time scales, respectively. From Fig.6(a) and 6(b), it can be seen that the pulse amplitudes are irregular and random distribution. Fig.6(c) shows a detailed view of the pulse, from which one can see that the pulse width and period are about 2.0 µs and 6.1 µs, respectively. Fig.6(d) shows the measured optical spectrum and the center wavelength is about 1 056 nm.

In order to judge whether the pulse amplitudes of the Nd:LMA laser are chaotic, we extract the peak values of pulses and reconstructed a PPTS as shown the red dots and lines in Fig.7(a) and 7 (b). Fig.7(a) and 7(b) represent the PPTS with 0.05 s and 0.000 2 s time scales, respectively. Further, for the reconstructed PPTS, the autocorrelation curve, phase portrait, power spectrum, and stochastic histogram are calculated and analyzed. Fig.7(c) shows the autocorrelation curve, and there is not apparent harmonic peak in the autocorrelation characteristics, which indicates the correlation of the PPTS is statistically insignificant. Fig.7(d) shows the corresponding phase portrait, which has no discernable structure and is a scattered distribution in a certain range. It reveals that there is no correlation between one pulse peak value at one round to the neighboring round and the trajectory in phase space move in a disordered form. The corresponding power spectrum is shown as Fig.7(e), which is approximately continuous frequency range in the power spectrum. In addition, the stochastic histogram of the PPTS is shown as Fig.7(f), which distribution is relatively symmetrical. Hence, the pulse amplitudes of the Nd:LMA laser is judged as chaos through the autocorrelation curve, phase portrait, power spectrum, and stochastic histogram of the PPTS. It is worth noting that the chaos characteristics in this paper are similar to those of a DPSS Q-switched Nd:YAG laser reported by TANG D Y, which demonstrates that the DPSS passively Q-switched laser is a typical chaotic dynamic system, and its dynamics are determined by low dimensional chaotic attractors[

3 Conclusion
In conclusion, by adopting a laser diode as a pump source, a Nd:LMA disordered crystal as a laser gain medium, a SESAM as a saturable absorber, a diode-pumped Nd:LMA pulsed laser are experimentally demonstrated. In the experimental system, the Nd:LMA pulsed laser can operate at Q-switched state or PAC state depended on the pump power, where the chaos of the PAC laser is proved through extracting by the autocorrelation curve, phase portrait, power spectrum, and stochastic histogram of the pulse-peak time series. When the pump power is in the range of 4.8~8.6 W, the Nd:LMA laser operates at a Q-switched state, and the repetition frequencies and pulse widths are in the range of 114.6~157.1 kHz and 4.0~2.2 µs, respectively. When the pump power is in the range of 8.7~10.5 W, the Nd:LMA laser operates at a PAC state. For a pump power of 10.5 W, the average output power of the PAC laser arrives at about 814 mW. However, the power of chaotic laser based on semiconductor lasers is only a few milliwatts. Therefore, this new experimental scheme for generating chaotic laser based on diode-pumped solid-state lasers may be a potential method for acquiring chaotic laser with high power.
References
[1] C S MONTROSS, T WEI, L YE. Laser shock processing and its effects on microstructure and properties of metal alloys: a review. International Journal of Fatigue, 24, 1021-1036(2002).
[2] U KELLER. Recent developments in compact ultrafast lasers. Nature, 424, 831-838(2003).
[3] P K UPPUTURI, M PRAMANIK. Performance characterization of low-cost, high-speed, portable pulsed laser diode photoacoustic tomography (PLD-PAT) system. Biomedical Optics Express, 6, 4118-4129(2015).
[4] U KELLER, D A B MILLER, G D BOYD. Solid-state low-loss intracavity saturable absorber for Nd:YLF lasers: an antiresonant semiconductor Fabry-Perot saturable absorber. Optics Letters, 17, 505-507(1992).
[5] U KELLER, K J WEINGARTEN, F X KÄRTNER. Semiconductor saturable absorber mirrors (SESAM's) for femtosecond to nanosecond pulse generation in solid-state lasers. IEEE Journal of Selected Topics in Quantum Electronics, 2, 435-453(1996).
[6] MEN Shaojie, Zhaojun LIU, Zhenhua CONG. Synchronized dual tunable wavelength Q-switched Nd:glass laser. Optics Express, 22, 30865-30872(2014).
[7] M LIU, J LIU, L LI. Experimental study on pulse characteristics of diode-pumped Q-switched Nd:KGW laser. Laser Physics Letters, 6, 437-440(2009).
[8] A MALEKI, M KAVOSH TEHRANI, H SAGHAFIFAR. Experimental study of electro-optical Q-switched pulsed Nd:YAG laser. Chinese Physics B, 25, 034206(2016).
[9] Jian MA, Tingting LU, Xiaolei ZHU. 1.57 MW peak power pulses generated by a diode-pumped Q-switched Nd:LuAG ceramic laser. Chinese Optics Letters, 15, 121402(2017).
[10] Qianqian HAO, Siyuan PANG, Jie LIU. Tunable and passively Q-switched laser operation of Nd,Lu:CaF2 disordered crystal. Applied Optics, 57, 6491-6495(2018).
[11] Nana ZHANG, Xiaodong XU, Yuxin PAN. Broadband spectra and efficient laser performance of a disordered Nd:Sr0.7La0.3Mg0.3Al11.7O19 crystal. Materials, 11, 1575(2018).
[12] L D SCHEARER, M LEDUC, D VIVIEN. LNA: a new CW Nd laser tunable around 1.05 and 1.08 µm. QE-, 22, 713-717(1986).
[13] T Y FAN, M R KOKTA. End-pumped Nd:LaF3 and Nd:LaMgAl11O19 lasers. IEEE Journal of Quantum Electronics, 25, 1845-1849(1989).
[14] J HAMEL, A CASSIMI, H ABU-SAFIA. Diode pumping of LNA lasers for helium optical pumping. Optics Communications, 63, 114-117(1987).
[15] Xiurong ZHANG, Xinmin ZHANG, Jun XU. Laser properties of lamp pumped La1-xMgNdxAl11O9 crystals. Chinese Journal of Lasers, 19, 496-498(1992).
[16] Zhenhong JIN, Jiang LU, Zhaoming HUANG. LDA pumped slab Nd:LMA laser. Laser Journal, 15, 97-101(1994).
[17] Hongru YANG, Xinglong YAN, Shuicai WANG. CW output in diode pumped Nd:LMA laser operating at 1054 nm. Acta Photonica Sinica, 26, 282-284(1997).
[18] Hongru YANG, Xinglong YAN, Shuicai WANG. Study on CW output of diode pumped Nd:LMA laser. Acta Optical Sinica, 17, 1407-1409(1997).
[19] Hongru YANG, Xinglong YAN, Zenghu CHANG. Study on diode-pumped Kerr-lens mode-locked Nd:LMA laser. Acta Photonica Sinica, 25, 870(1996).
[20] Xiaohua SHEN, Chun WANG, Hongru YANG. Additive pulse mode locking of a Nd:LMA laser. Chinese Journal of Lasers, 25, 577-580(1998).
[21] Yuxin PAN, Shidong ZHOU, Jiawei WANG. Growth, spectral properties, and diode-pumped laser operation of a Nd3+-doped LaMgAl11O19 crystal. Applied Optics, 57, 9657-9661(2018).
[22] Jiawei WANG, Yuzhao ZHANG, Xiaofeng GUAN. High-efficiency diode-pumped continuous-wave and passively Q-switched c-cut Nd:LaMgAl11O19 (Nd:LMA) lasers. Optics Materials, 86, 512-516(2018).
[23] Pu LI, Yuncai WANG, Anbang WANG. Direct generation of all-optical random numbers from optical pulse amplitude chaos. Optics Express, 20, 4297-4308(2012).
[24] D Y TANG, L J QIN. Deterministic chaos in a diode-pumped Nd:YAG laser passively Q switched by a Cr4+:YAG crystal. Optics Letters, 28, 325-327(2003).
[25] Yanhua HONG, J PAUL. GHz bandwidth message transmission using chaotic vertical-cavity surface-emitting lasers. Journal of Lightwave Technolonogy, 27, 5099-5105(2009).
[26] Lilin YI, Junxiang KE. Research progress of chaotic secure optical communication. Journal of Communications, 41, 168-181(2020).
[27] F Y LIN, J M LIU. IEEE Journal of Quantum Electronics, 40, 815-820(2004).
[28] Xiaozhou LI, Songsui LI, Szechun CHAN. Correlated random bit generation using chaotic semiconductor lasers under unidirectional optical injection. IEEE Photonics Journal, 9, 1505411(2017).
[29] Shuiying XIANG, Wei PAN, Nianqiang LI. Randomness-enhanced chaotic source with dual-path injection from a single master laser. IEEE Photonics Technology Letters, 24, 1753-1756(2012).
[30] D BRUNNER, M C SORIANO, C R MIRASSO. Parallel photonic information processing at gigabyte per second data rates using transient states. Nature Communications, 4, 1364(2013).
[31] Yushuang HOU, Guangqiong XIA, Wenyan YANG. Prediction performance of reservoir computing system based on a semiconductor laser subject to double optical feedback and optical injection. Optics Express, 26, 10211-10219(2018).
[32] Yihuan LIAO, Fanyi LIN. Dynamical characteristics and their applications of semiconductor lasers subject to both optical injection and optical feedback. Optics Express, 21, 23568-23578(2013).
[33] Dongzhou ZHONG, Yongqiang JI, Wei LUO. Controllable optoelectric composite logic gates based on the polarization switching in an optically injected VCSEL. Optics Express, 23, 29823-29833(2015).
[34] Xiaozhou LI, Songsui LI, Szechan CHAN. Correlated random bit generation using chaotic semiconductor lasers under unidirectional optical injection. IEEE Photonics Journal, 9, 1505411(2017).
[35] Tao DENG, Guangqiong XIA, Jianjun CHEN. Experimental investigation on nonlinear dynamics of 1550nm VCSEL simultaneously subject to orthogonal optical injection and negative optoelectronic feedback. Laser Physics, 27, 045402(2017).
[36] Jianjun CHEN, Zhengmao WU, Tao DENG. Current- and feedback-induced state bistability in a 1550nm-VCSEL with negative optoelectronic feedback. IEEE Photonics Journal, 9, 1500310(2017).
[37] R SAKURABA, K IWAKAWA, K KANNO. Tb/s physical random bit generation with bandwidth-enhanced chaos in three-cascaded semiconductor lasers. Optics Express, 23, 1470-1490(2015).
[38] G BOUCHEZ, B MACIAS. Wideband chaos from a laser diode with phase-conjugate feedback. Optics Letters, 44, 975-978(2019).
[39] Lijun QIAO, Tianshuang LV, Yong XU. Generation of flat wideband chaos based on mutual injection of semiconductor lasers. Optics Letters, 44, 5394-5397(2019).
[40] Songsui LI, Xiaozhou LI, Szechun CHAN. Chaotic time-delay signature suppression with bandwidth broadening by fiber propagation. Optics Letters, 43, 4751-4754(2018).
[41] Yining ZHANG, Aishi XU, Yuling FENG. Chaos characteristics of the output from a semiconductor laser subject to optoelectronic feedback. Acta Optica Sinica, 40, 1214001(2020).
[42] Xin YANG, Jianjun CHEN, Zhengmao WU. Nonlinear dynamics of 1550 nm vertical-cavity surface-emitting lasers subject to negative optoelectronic feedback. Acta Photonica Sinica, 45, 0814004(2016).
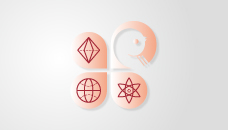
Set citation alerts for the article
Please enter your email address