Qingzhe Gou, Ning Wei, Haixu Tao, Kang Cheng, Yongtao Fan, Jiajing He, Jun Wang, "Miniature laser frequency stabilization module for cold atom sensing," Chin. Opt. Lett. 22, 101302 (2024)

Search by keywords or author
- Chinese Optics Letters
- Vol. 22, Issue 10, 101302 (2024)
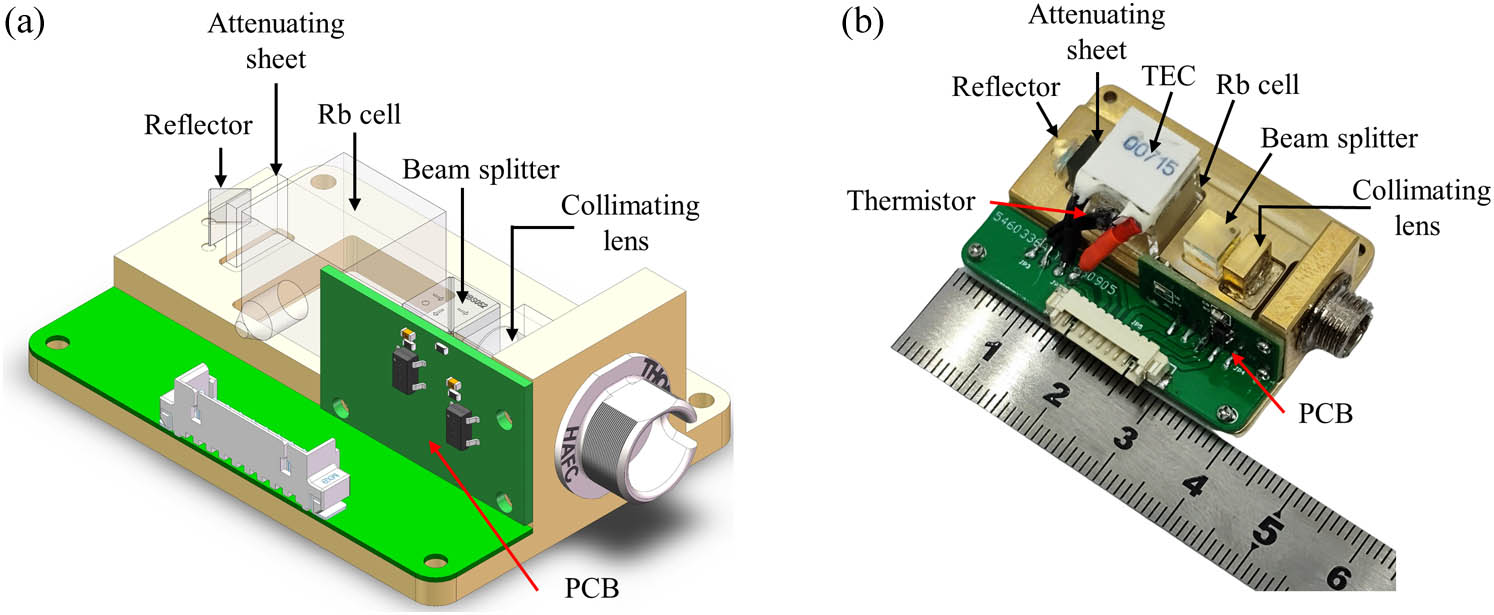
Fig. 1. Structure of the miniature laser frequency-stabilization module. (a) Three-dimensional image of the miniature frequency-stabilization module; (b) photograph of the module after assembly. PCB, printed circuit board; TEC, thermoelectric cooler.

Fig. 2. Hyperfine structure of 85Rb and 87Rb D2 line.

Fig. 3. Block diagram of the miniature laser frequency-stabilization module. The two solid line boxes represent the components packaged by the module—optical and electrical parts, respectively, and the dashed line box represents the components of the digital control circuits utilized in the feedback loop.

Fig. 4. (a) Signal absorption of Rb bubble saturation with a length of 10 and 50 mm; (b) Fg = 2 → Fe = 1 error signal for 87Rb 52S1/2 → 52P3/2 transition.

Fig. 5. Saturation absorption spectra of Rb cell at different temperatures.

Fig. 6. Output laser frequency fluctuates over 1000 s under free-running and locked conditions. The black curve before the 1000 s shows the frequency drift range of the free-running 780 nm laser is 22 MHz. After the feedback is on, the red and gray lines show the more than 20 times reduction of the frequency drift of the 780 nm laser locked to the miniature module and the commercial stabilization setup, respectively.

Fig. 7. (a) Magneto-optical traps; (b) cloud of cold atoms.
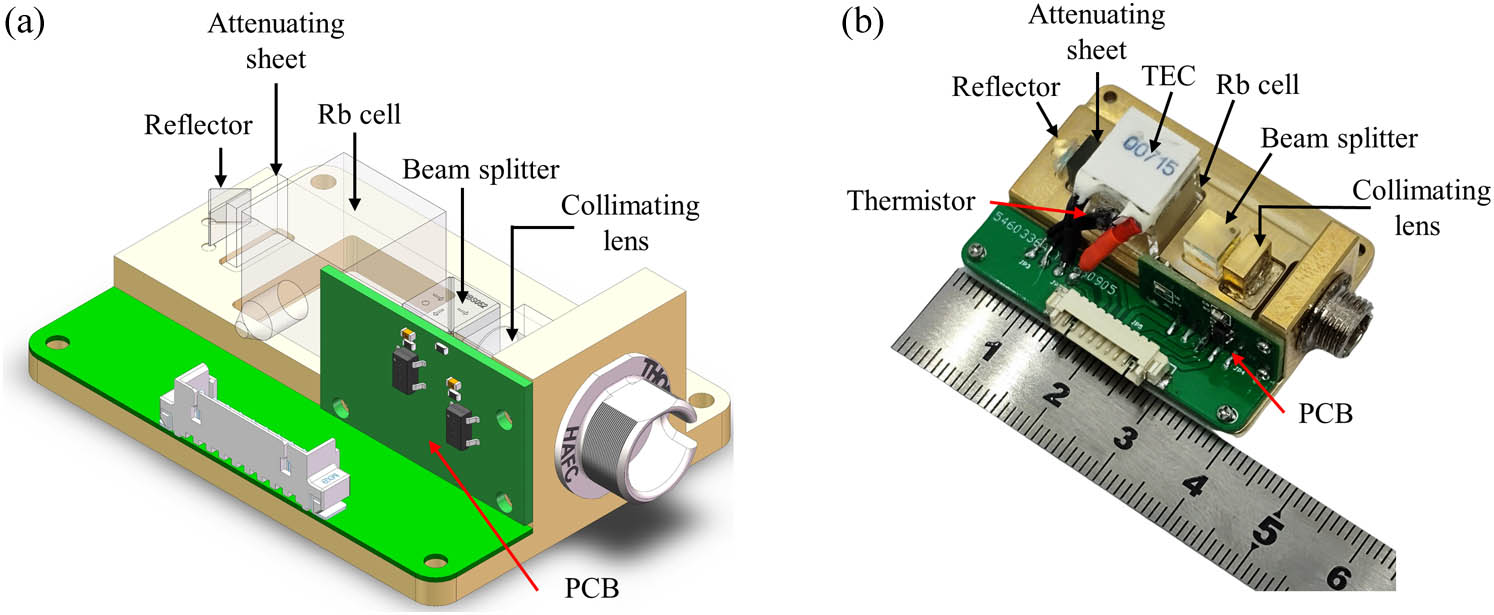
Set citation alerts for the article
Please enter your email address