Wenliang Zhang, Onur Çakıroğlu, Abdullah Al-Enizi, Ayman Nafady, Xuetao Gan, Xiaohua Ma, Sruthi Kuriakose, Yong Xie, Andres Castellanos-Gomez. [J]. Opto-Electronic Advances, 2023, 6(3): 220101

Search by keywords or author
- Opto-Electronic Advances
- Vol. 6, Issue 3, 220101 (2023)
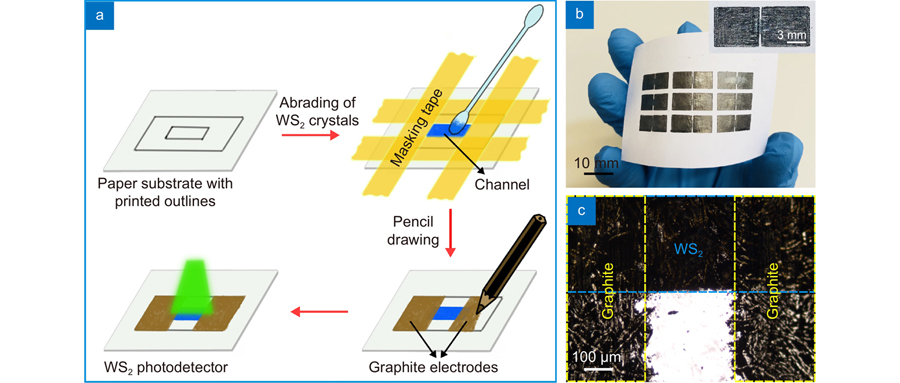
Fig. 1. (a ) Schematic illustration of the fabrication process of paper-based WS2 photodetectors via abrading WS2 crystals and penciling graphite electrodes on paper substrates. (b ) Photograph of the 3 × 3 WS2 photodetector array. Inset shows the magnified view of a WS2 photodetector. (c ) Optical micrograph of a WS2 photodetector showing the WS2 channel and graphite electrode regions.

Fig. 2. Comparison of the photoresponse performance of the paper-based WS2 photodetector (device A) tested in air and vacuum conditions under illumination. (a ) Current vs. time across the device under a periodic ON/OFF switching of illumination with a power intensity of 35 mW cm−2. (b ) Zoomed in on three consecutive ON/OFF cycles from (a). (c ) Photocurrent vs. time for the WS2 device as the illumination is switched ON/OFF with increasing incident power intensity from 1.1 mW cm−2 to 35 mW cm−2. (d ) Photocurrent as a function of the power intensity. Note: Measurements are carried out at a bias voltage of 10 V and with a selected wavelength of 617 nm. The channel length and width of the device A are ~300 μm and 2 mm, respectively.

Fig. 3. Voltage-dependent photoresponse of the paper-based WS2 photodetector (device B) under the illumination of 617 nm. (a ) Photocurrent as a function of time for the WS2 photodetector while the light is switched ON/OFF under various power intensities at a fixed bias voltage of 35 V. (b ) The measured photocurrent and (c ) corresponding responsivity as a function of power intensity collected at various bias voltages from 1 to 35 V. (d ) The measured photocurrent and responsivity as a function of bias voltage at a fixed power intensity of 35 mW cm−2.

Fig. 4. Spectral response of the paper-based WS2 photodetector (device B). (a ) Photocurrent vs. time when the device is subjected to cycles of ON/OFF illumination with different wavelengths. (b ) Spectrum response of the WS2 photodetector under various wavelengths of illumination in the range of 365 nm (ultraviolet) to 940 nm (near-infrared). Note: The device is measured at a fixed voltage of 10 V and an incident power intensity of 13 mW cm−2.

Fig. 5. Integration of the paper-based WS2 photodetector as detection element in an optical spectrometer. (a ) Schematic diagram of the spectrometer system consist of a light source, a light-scattering optical element (reflective diffraction grating), and a detection element. (b ) The measured power profiles using a commercial silicon photodiode and (c ) photocurrent profiles using the paper-based WS2 photodetector (device C). Note: As light source we have used a supercontinuum laser with different spectral filters.
|
Table 1. Comparison of typical device characteristics of the present WS2 in this work and other TMDCs-based and paper-supported photodetection devices. Response time values highlighted with r or f represent the rise time and fall time values, respectively.
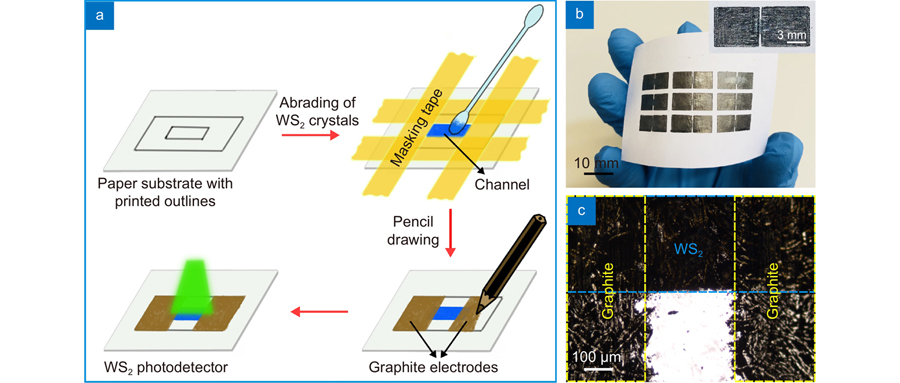
Set citation alerts for the article
Please enter your email address