Naijun Cheng, Weifan Li, Feng Qi. Progress of Mid-Infrared Laser[J]. Laser & Optoelectronics Progress, 2023, 60(17): 1700006

Search by keywords or author
- Laser & Optoelectronics Progress
- Vol. 60, Issue 17, 1700006 (2023)
![Structure diagram of combustion-driven continuous wave HF/DF chemical laser[6]](/richHtml/lop/2023/60/17/1700006/img_01.jpg)
Fig. 1. Structure diagram of combustion-driven continuous wave HF/DF chemical laser[6]
![System composition of an electrically excited chemical laser[6]](/richHtml/lop/2023/60/17/1700006/img_02.jpg)
Fig. 2. System composition of an electrically excited chemical laser[6]
![HBr laser output spectrum and laser output power curve[10]](/Images/icon/loading.gif)
Fig. 3. HBr laser output spectrum and laser output power curve[10]
![Diagram of fiber gas laser based on population inversion[16]](/Images/icon/loading.gif)
Fig. 4. Diagram of fiber gas laser based on population inversion[16]
![Single-pass configuration experiment of fiber acetylene gas CW laser output[20]. (a) Diagram of experimental setup; (b) output laser power as a function of absorbed pump powers at different pressures](/Images/icon/loading.gif)
Fig. 5. Single-pass configuration experiment of fiber acetylene gas CW laser output[20]. (a) Diagram of experimental setup; (b) output laser power as a function of absorbed pump powers at different pressures
![Experiment of OPO pumping CO2-filled silver plating capillary[21]. (a) Diagram of experimental setup; (b) output spectrum and energy level transition principle](/Images/icon/loading.gif)
Fig. 6. Experiment of OPO pumping CO2-filled silver plating capillary[21]. (a) Diagram of experimental setup; (b) output spectrum and energy level transition principle
![Output characteristics of fiber acetylene gas laser[24]. (a) Laser output spectra under different signal powers at 300 Pa pressure; (b) signal power versus pump power at 300 Pa pressure with output light field shown in inset](/Images/icon/loading.gif)
Fig. 7. Output characteristics of fiber acetylene gas laser[24]. (a) Laser output spectra under different signal powers at 300 Pa pressure; (b) signal power versus pump power at 300 Pa pressure with output light field shown in inset
![Schematic diagrams of energy level transitions of Tm3+, Ho3+ and Er3+(from left to right)[29]](/Images/icon/loading.gif)
Fig. 8. Schematic diagrams of energy level transitions of Tm3+, Ho3+ and Er3+(from left to right)[29]
![Overall experimental scheme[36]. (a) Energy level diagram of GSA and ESA dual-wavelength pumped scheme; (b) experimental arrangement for GSA and ESA dual-wavelength pumped Tm3+∶YAP laser](/Images/icon/loading.gif)
Fig. 9. Overall experimental scheme[36]. (a) Energy level diagram of GSA and ESA dual-wavelength pumped scheme; (b) experimental arrangement for GSA and ESA dual-wavelength pumped Tm3+∶YAP laser
![Configuration of tunable multi-wavelength Ho3+ doped fiber laser[49]](/Images/icon/loading.gif)
Fig. 10. Configuration of tunable multi-wavelength Ho3+ doped fiber laser[49]
![Diagrams of side-pumped Er3+∶YSGG slab laser; (a) Top view; (b) side view[52]](/Images/icon/loading.gif)
Fig. 11. Diagrams of side-pumped Er3+∶YSGG slab laser; (a) Top view; (b) side view[52]
![Schematic diagram of 140 W Cr2+∶ZnSe laser system[67]](/Images/icon/loading.gif)
Fig. 12. Schematic diagram of 140 W Cr2+∶ZnSe laser system[67]
![Joule level Fe2+∶ZnSe mid-IR laser pumped by Er3+∶YAG lasers[69]](/Images/icon/loading.gif)
Fig. 13. Joule level Fe2+∶ZnSe mid-IR laser pumped by Er3+∶YAG lasers[69]
![30.6 mJ, Fe2+∶ZnSe mid-IR laser pumped by HF laser operating at room temperature[70]](/Images/icon/loading.gif)
Fig. 14. 30.6 mJ, Fe2+∶ZnSe mid-IR laser pumped by HF laser operating at room temperature[70]

Fig. 15. Schematic diagram of band structure of quantum cascade laser
![Schematic diagram of experimental apparatus for polarization beam combination[86]](/Images/icon/loading.gif)
Fig. 16. Schematic diagram of experimental apparatus for polarization beam combination[86]
![Schematic diagram of conversion process under several nonlinear frequencies[87]](/Images/icon/loading.gif)
Fig. 17. Schematic diagram of conversion process under several nonlinear frequencies[87]
![Schematic diagram of violet jade laser pumped AgGaS2 and GaSe MIR-DFG[89]](/Images/icon/loading.gif)
Fig. 18. Schematic diagram of violet jade laser pumped AgGaS2 and GaSe MIR-DFG[89]
![Schematic diagram of MIR source based on ps-laser pumped BaGa4Se7 crystal[94]](/Images/icon/loading.gif)
Fig. 19. Schematic diagram of MIR source based on ps-laser pumped BaGa4Se7 crystal[94]
![Schematic diagram of CW MIR source based on BaGa4Se7-DFG[96]](/Images/icon/loading.gif)
Fig. 20. Schematic diagram of CW MIR source based on BaGa4Se7-DFG[96]
![PPLN-OPO structure diagram[100]](/Images/icon/loading.gif)
Fig. 21. PPLN-OPO structure diagram[100]
![MgO∶PPLN-OPO experimental apparatus[104]](/Images/icon/loading.gif)
Fig. 22. MgO∶PPLN-OPO experimental apparatus[104]
![Experiment of mid-infrared laser source based on ZnGeP2-OPO; (a) Tm3+-doped fiber+Ho3+∶YAG rod pumped ZGP-OPO mid-infrared laser[109]; (b) based on Rb∶PPKTP pumped mid-infrared ZnGeP2-OPO[111]](/Images/icon/loading.gif)
Fig. 23. Experiment of mid-infrared laser source based on ZnGeP2-OPO; (a) Tm -doped fiber+Ho3+∶YAG rod pumped ZGP-OPO mid-infrared laser[109]; (b) based on Rb∶PPKTP pumped mid-infrared ZnGeP2-OPO[111]
|
Table 1. Research progress of HCF based on population inversion [16]
|
Table 2. Tuning range and width of Tm3+ doped laser with different substrates[30]
|
Table 3. Research progress of Tm3+ solid-state laser
|
Table 4. Optical properties of some infrared nonlinear crystals[87]
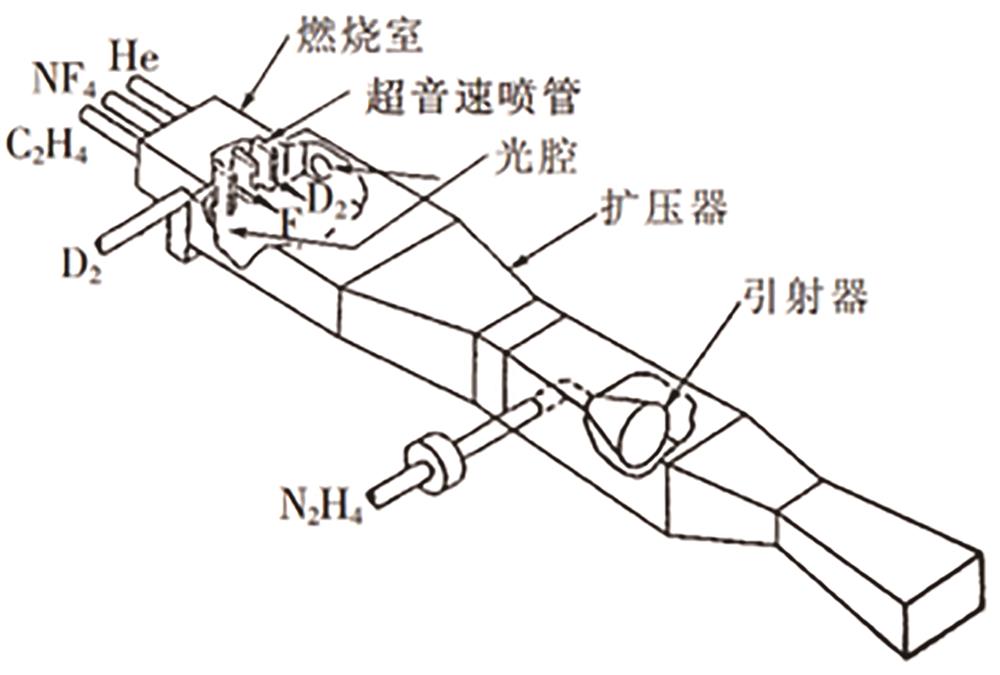
Set citation alerts for the article
Please enter your email address