
- Opto-Electronic Advances
- Vol. 7, Issue 9, 240040-1 (2024)
Abstract
Introduction
In the era of fifth-generation (5G) wireless communications where devices are heavily used, due to the great advances in the Internet of Things (IoT) and artificial intelligence (AI), perceivable devices with miniaturization and flexible regulations are facilitated for managing the wireless channels, and many interesting applications are therefore developed. Hence, in the physical level, the wireless channel and electromagnetic (EM) link between the transmitter and receiver with complex propagation internally play an important role in the future wireless communications.
On the other hand, surface plasmon polariton (SPP) in the optical frequency region
Specifically, many excellent characteristics of SSPP electromagnetic materials such as flexible design, greater slow-wave, EM coupling, low cost, low loss, low profile, etc., make it more conducive to the design of antennas, which can control the near field by the control unit arrangement to change the radiation effect in the far field. For example, as one type of beam-scanning antennas (BSA), leaky-wave antennas (LWA) with periodic radiation elements or continuous apertures
Hence, the reconfigurable SSPP-LWA is very suitable for developing a new type of smart antenna because of the outstanding ability of dispersion regulation and high freedom of wave control. The field of smart antenna is constantly evolving, generally including the following three categories. First, beamforming antennas
In this paper, we propose a new approach combining reconfigurable SSPP-LWA with CV as a smart antenna system to realize the global management of wireless channels in the process of transmission, and automatically meet the needs of users in dynamic environment. By adding PIN diodes on the radiating element and adjusting the bias voltage of the PIN diode, the working state (e.g., the radiating state and the non-radiating state) is controlled dynamically within the frequency band. Experiments show that in the “radiating” state, the beam scanning range of the reconfigurable SSPP-LWA is −43°~5° in the frequency band from 8.5 to 13 GHz. Next, visual information is employed to assist SSPP-LWA in adjusting beam scanning. The proposed vision-based scheme utilizes advanced CV technology to acquire the position information of the user relative to the reconfigurable SSPP-LWA, sends the position information to the computer, and then feedbacks instructions to the FPGA and signal generator. We provide two experiment scenarios. In the first one, the camera is used to detect whether there is a target in the specified range, and the adaptive real-time switching between the radiation state and the non-radiation state is realized accordingly. In the second scenario, the SSPP-LWA provides feedback on the base station side according to the target position, and supplies the desired frequency instruction in line with the direction towards the selected target, thereby realizing beam tracking.
The proposed reconfigurable SSPP-LWA combined with CV can perceive the moving targets through target detection, and then dynamically adjust the use of spectrum resources. In wide-band communication, the SSPP-LWA can adjust the operating frequency according to this information to avoid spectrum conflict and improve spectrum utilization. In the optimization of energy efficiency, combined with the real-time perception of CV, the smart SSPP-LWA can more intelligently select the operating mode and frequency in wide-band communication to optimize the energy efficiency of the communication system, reduce power consumption. Also, the smart SSPP-LWA can switch the antenna working state according to the presence of the target and realize target beam tracking in real time, enhance physical layer communication security.
Results and discussion
Architecture of the intelligent scheme
The schematic of the proposed intelligent system is presented in
Figure 1.
Figure 2.
The moving object is tracked dynamically and its position information is refreshed in real-time by the RS-Camera, with each refresh followed by a voltage control sequence that feeds the FPGA or by the corresponding frequency signal that feeds the signal generator connected to the reconfigurable SSPP-LWA. The input voltage and frequency of the reconfigurable SSPP-LWA is obtained through the computer using the feedback information, and the instruction are constantly refreshed. In the first experimental scenario, by the voltage that feedback from detecting whether there are external objects within a specific range, the smart SSPPs LWA can realize the adaptive real-time switching between the radiation state and the non-radiation state. In the second experimental scenario, the smart SSPP-LWA is carefully designed to transmit a frequency-dependent scanning beam towards the moving target with regard to its changing positions. Among them, the coordinate systems of the camera and the reconfigurable SSPP-LWA (as they are closely located) are unified to ensure the accuracy of the position.
Functional demonstrations of the reconfigurable SSPP-LWA
Theory of radiation
The theoretical analysis in this section includes two parts, one is reconfiguration of state switching in the operating frequency band, and the other is frequency-controlled beam scanning of the SSPP-LWA when PIN diode is turned on.
Firstly, the radiation principle of the SSPP-LWA is analyzed. The dispersion curve of SSPPs TL is taken as the starting point, as shown in
Figure 3.
where kspp is the propagation constant of the n = 0 harmonic (the fundamental mode), and d is the distance between two adjacent radiation unit cells. In
The detailed structure of reconfigurable SSPP-LWA is shown in
Figure 4.(
The switchable radiating/non-radiating working state is caused by the change of the state of the PIN diodes embedded in the radiating patch, which are mounted on either side of the ELC resonant structure, as shown in
The switching of the working state of the antenna is controlled by the bias voltage of the PIN diode. When the PIN diode is turned on, the antenna is in the radiating state; and when it is turned off, the antenna is in the transmission (non-radiating) state. In the topology design of the PIN diode of the patch, the isolation of switching states and the working bandwidth should be weighed. Series switches have the advantage of low transmission loss in broadband but at the price of low isolation. Parallel switches are usually used in combination with quarter-wavelength TLs, which are narrow in nature, but have higher isolation than series switches. In addition, using two or more PIN diodes in series mode can improve the isolation of one PIN diode, and the same bias current can be used in series connection to save power. In this work, in order to realize broadband performance, high isolation and low-power dissipation, two PIN diodes are designed in series. (Please see Supplementary information Section 4 for the equivalent circuit of the PIN diode used).
Toggle of transmission and radiation
The reconfigurable SSPP-LWA redistributes the current distribution on the antenna by switching the diode between “ON” and “OFF” states, and so as to control the coupling state between the radiation units and the SSPPs TL. The antenna adopts the SSPP TL based on “T”-shaped unit structure as the feed, uses periodically loads radiation units on both sides of the TL to stimulate the higher harmonic for radiation, and selects the −1 order harmonic for single mode radiation. The less-lossy dielectric substrate of the antenna is Taconic TLY-5 (εr = 2.2, tanδ = 0.0009) with a thickness of 0.762 mm, and the copper thickness is 0.018 mm. The overall size of the designed SSPP-LWA is 269×63.24 mm2.
The commercial software, Computer Simulation Technology (CST) Microwave Studio was used to conduct time-domain full-wave simulation of the above antenna.
Figure 5.
To verify the proposed model, we plot the simulated local current distribution of the coupling part and radiation unit in
There are also some similar studies on the principles of switching or modulating radiation and transmission of waves. Firstly, metasurfaces can convert spatially incident CP beams into spoof surface plasmons (SSP) with the same wave vector through phase gradients in the THz regime
In view of this, when the PIN diode is disconnected, the EM energy in the radiation unit does not radiate out, and most of the energy is transmitted, that is, the antenna changes from the state of leakage wave radiation to the state of transmission. In
Radiation demonstration of the reconfigurable SSPP-LWA
For more visualized perception of the beam scanning capability,
Figure 6.
To gain a more vivid understanding of the beam scanning capability, simulated results for the frequency scanning of reconfigurable SSPP-LWA with two working states are shown in
To verify the above theoretical analysis and demonstrate the good performance, the prototype of the proposed SSPP-LWA is fabricated and measured. The radiation patterns are tested in an anechoic chamber environment, as shown in
Figure 7.(
Perceptible platform based on reconfigurable SSPP-LWA and computer vision
In wireless communication, radar system can detect and track targets in the complex EM environment. However, due to the high cost, large size and complex design, other technologies are urgently needed to make up for it. Fortunately, with the fast development of computer vision technology, intuitive, reliable, and cost-effective target detection and tracking become possible in many application scenarios. Moreover, AI-enabled computer vision (CV) technology is evolving rapidly, which can solve more complicated problems and serve as an aid to intelligent communications. Here, it is designed as a perceptible innovative technology, with the proposed smart SSPP-LWA system to realize an intelligent perceptible platform that combines perception and communication.
Target detection based radiation switchable SSPP-LWA
The externally perceptive platform is shown in
Figure 8.
In the test scene, we use a toy car instead of a detector or jammer as the target, and the field of view in
It can be observed that the S-parameter is very consistent with the measured results in
Beam tracking of the SSPP-LWA with vision aid
We have carried out the intelligent system experiment of beam tracking. By detecting the location of the receiver (a horn antenna) with a RS-Camera, we can send the frequency instruction of the corresponding beam direction to the signal generator (the SSPP LWA), so as to realize the closed-loop operation of the system without human instruction. The system consists of a signal generator (SA, Keysight E8257D), a signal analyzer (SG), the SSPP-LWA, a RS-Camera, a computer and a receiver (a dual-ridged horn antenna, 750 MHz to 18 GHz). The SG is connected to the LWA, the receiver is connected to the SA, and the computer controls the RS-Camera and SG respectively, as shown in
Figure 9.(
Firstly, we tested the accepted energy values for all frequencies without the camera working. It can be seen that the receiving antenna is fixed on a slide rail and bisects ten moving points in a horizontal movement of 1.5 m. Each point position measures the received energy at different emission frequencies. The signal generator transmits 9–13 GHz EM waves and records them every 0.2 GHz. The task of the vision-aided SSPP-LWA is to accurately radiate the EM waves to the horn antenna at the receiver side based on the visual information. In test progress, as for the vision-based beam tracking scheme, we use object detection and CV technology to obtain the position information of the horn antennas with respect to the SSPP-LWA. Then beam tracking is implemented in two stages. In the first stage, since we have actually tested the radiation status of SSPP-LWA, we can determine the correspondence of the angle with regard to the frequency. In the second stage, according to the coordinates of the target user, SSPP-LWA can obtain the desired beam direction and select the corresponding codeword in the precoder to achieve beam tracking. We can get the direction of the predicted bounding box of the target user at a certain moment through the visual algorithm, as shown in
During testing, we move the target user within coverage areas that include SSPP-LWA beamforming. To highlight the effectiveness of vision, we plot in one diagram with visual aids and in all situations. When there is no visual aid, the traditional frequency scanning method, in which the feedback procedure is ignored, traverses the received power at each frequency point at all locations. When there is visual assistance, the strategy of beam tracking is: first use RS-Camera to feedback the position of the target user in the field of view, and when the user is outside the beam scanning range, the computer does not send instructions to the SG. And when the user is within the coverage range of SSPP-LWA beamforming, the computer sends the frequency instruction of the response to SG according to the returned angle information. The SSPP-LWA’s operating frequency is adjusted from 8.5 GHz to 13.1 GHz to allow the platform to operate at a sufficient scanning range. It can be seen that the blue five-star mark records the receiving power value of different positions in
Figure 10.The received power of the target user corresponds to different frequencies during the movement. The blue pentagon is marked as the received power of the target user with vision aid.
At the same time, there is no additional feedback process and beam training overhead throughout the communication. For traditional passive devices, it is basically difficult to achieve beam tracking and dynamic switching. The smart system adjusts the operating frequency according to the information of target detection in the process of beam scanning to avoid spectrum conflict, improve spectrum utilization, and reduce overhead.
Conclusions
In this paper, we propose a smart SSPP-LWA with external perceptibility, which can realize the adaptive real-time switching between the radiation state and the non-radiation state and beam tracking by regulating the coupling unit. In two intelligent communication scenarios, the SSPP-LWA obtains the real-time location information of the external jammer or target user through the CV, switches the working states accordingly, and realizes automatic tracking of moving target by adjusting the input frequency. Leveraging the capabilities of beam reconfiguration and external perception, the proposed SSPP-LWA serves as a prime contender for smart systems, offering the ability to autonomously select optimal operating modes and intelligent communication in dynamic, complex environments.
References
[1] WL Barnes, A Dereux, TW Ebbesen. Surface plasmon subwavelength optics. Nature, 424, 824-830(2003).
[2] FJ Garcia-Vidal, L Martín-Moreno, JB Pendry. Surfaces with holes in them: new plasmonic metamaterials. J Opt A Pure Appl Opt, 7, S97-S101(2005).
[3] QQ Gan, Z Fu, YJ Ding et al. Ultrawide-bandwidth slow-light system based on THz plasmonic graded metallic grating structures. Phys Rev Lett, 100, 256803(2008).
[4] XP Shen, TJ Cui, D Martin-Cano et al. Conformal surface plasmons propagating on ultrathin and flexible films. Proc Natl Acad Sci USA, 110, 40-45(2013).
[5] HF Ma, XP Shen, Q Cheng et al. Broadband and high-efficiency conversion from guided waves to spoof surface plasmon polaritons. Laser Photon Rev, 8, 146-151(2014).
[6] A Kianinejad, ZN Chen, CW Qiu. Low-loss spoof surface plasmon slow-wave transmission lines with compact transition and high isolation. IEEE Trans Microw Theory Tech, 64, 3078-3086(2016).
[7] PH He, HC Zhang, XX Gao et al. A novel spoof surface plasmon polariton structure to reach ultra-strong field confinements. Opto-Electron Adv, 2, 190001(2019).
[8] M Wang, S Sun, HF Ma et al. Supercompact and ultrawideband surface plasmonic bandpass filter. IEEE Trans Microw Theory Tech, 68, 732-740(2020).
[9] LF Ye, Y Chen, ZY Wang et al. Compact spoof surface plasmon polariton waveguides and notch filters based on meander-strip units. IEEE Photonics Technol Lett, 33, 135-138(2021).
[10] YQ Liu, KD Xu, JX Li et al. Millimeter-wave E-plane waveguide bandpass filters based on spoof surface plasmon polaritons. IEEE Trans Microw Theory Tech, 70, 4399-4409(2022).
[11] XY Liu, Y Lei, X Zheng et al. Reconfigurable spoof plasmonic coupler for dynamic switching between forward and backward propagations. Adv Mater Technol, 7, 2200129(2022).
[12] LY Niu, PH He, Y Fan et al. Gain‐associated nonlinear phenomenon in single‐conductor odd‐mode plasmonic metamaterials. Laser Photon Rev, 16, 2100619(2022).
[13] XX Gao, JJ Zhang, Y Luo et al. Reconfigurable parametric amplifications of spoof surface plasmons. Adv Sci, 8, 2100795(2021).
[14] LL Liu, L Wu, JJ Zhang et al. Backward phase matching for second harmonic generation in negative-index conformal surface plasmonic metamaterials. Adv Sci, 5, 1800661(2018).
[15] J Zhang, HC Zhang, XX Gao et al. Integrated spoof plasmonic circuits. Sci Bull, 64, 843-855(2019).
[16] HC Zhang, LP Zhang, PH He et al. A plasmonic route for the integrated wireless communication of subdiffraction-limited signals. Light Sci Appl, 9, 113(2020).
[17] WX Tang, HC Zhang, HF Ma et al. Concept, theory, design, and applications of spoof surface plasmon polaritons at microwave frequencies. Adv Opt Mater, 7, 1800421(2019).
[18] MB Pu, XL Ma, YH Guo et al. Theory of microscopic meta-surface waves based on catenary optical fields and dispersion. Opt Express, 26, 19555-19562(2018).
[19] YH Guo, ZJ Zhang, MB Pu et al. Spoof plasmonic metasurfaces with catenary dispersion for two-dimensional wide-angle focusing and imaging. iScience, 21, 145-156(2019).
[20] XG Luo, MB Pu, YH Guo et al. Catenary functions meet electromagnetic waves: opportunities and promises. Adv Opt Mater, 8, 2001194(2020).
[21] JL Gomez-Tornero, FD Quesada-Pereira, A Alvarez-Melcon. Analysis and design of periodic leaky-wave antennas for the millimeter waveband in hybrid waveguide-planar technology. IEEE Trans Antennas Propag, 53, 2834-2842(2005).
[22] DR Jackson, C Caloz, T Itoh. Leaky-wave antennas. Proc IEEE, 100, 2194-2206(2012).
[23] M Wang, HF Ma, WX Tang et al. A dual-band electronic-scanning leaky-wave antenna based on a corrugated microstrip line. IEEE Trans Antennas Propag, 67, 3433-3438(2019).
[24] TR Cameron, GV Eleftheriades. Experimental validation of a wideband metasurface for wide-angle scanning leaky-wave antennas. IEEE Trans Antennas Propag, 65, 5245-5256(2017).
[25] AJ Mackay, GV Eleftheriades. Meandered and dispersion-enhanced planar leaky-wave antenna with fast beam scanning. IEEE Antennas Wirel Propag Lett, 20, 1596-1600(2021).
[26] DK Karmokar, KP Esselle, TS Bird. Wideband microstrip leaky-wave antennas with two symmetrical side beams for simultaneous dual-beam scanning. IEEE Trans Antennas Propag, 64, 1262-1269(2016).
[27] HY Chen, H Ma, YF Li et al. Wideband frequency scanning spoof surface plasmon polariton planar antenna based on transmissive phase gradient metasurface. IEEE Antennas Wirel Propag Lett, 17, 463-467(2018).
[28] J Wang, L Zhao, ZC Hao et al. Wide-angle frequency beam scanning antenna based on the higher-order modes of spoof surface plasmon polariton. IEEE Trans Antennas Propag, 68, 7652-7657(2020).
[29] SK Ge, QF Zhang, AK Rashid et al. Analysis of asymmetrically corrugated goubau-line antenna for endfire radiation. IEEE Trans Antennas Propag, 67, 7133-7138(2019).
[30] HR Zu, B Wu, YT Zhao et al. Dual-band antenna with large beam steering angle incorporating endfire and frequency scanning modes using double-layer SSPPs structure. IEEE Trans Antennas Propag, 70, 46-55(2022).
[31] A Sarkar, S Lim. Annular surface plasmon polariton-based frequency-scanning leaky-wave antenna for full azimuth coverage. IEEE Trans Antennas Propag, 70, 180-188(2022).
[32] JY Yin, J Ren, Q Zhang et al. Frequency-controlled broad-angle beam scanning of patch array fed by spoof surface plasmon polaritons. IEEE Trans Antennas Propag, 64, 5181-5189(2016).
[33] G Zhang, QF Zhang, YF Chen et al. High-scanning-rate and wide-angle leaky-wave antennas based on glide-symmetry goubau line. IEEE Trans Antennas Propag, 68, 2531-2540(2020).
[34] JY Lau, SV Hum. Reconfigurable transmitarray design approaches for beamforming applications. IEEE Trans Antennas Propag, 60, 5679-5689(2012).
[35] W Hong, ZH Jiang, C Yu et al. Multibeam antenna technologies for 5G wireless communications. IEEE Trans Antennas Propag, 65, 6231-6249(2017).
[36] I Ahmed, H Khammari, A Shahid et al. A survey on hybrid beamforming techniques in 5G: architecture and system model perspectives. IEEE Commun Surv Tutorials, 20, 3060-3097(2018).
[37] DX Zhao, P Gu, JC Zhong et al. Millimeter-wave integrated phased arrays. IEEE Trans Circuits Syst I Regul Pap, 68, 3977-3990(2021).
[38] TS Rappaport, F Gutierrez, E Ben-Dor et al. Broadband millimeter-wave propagation measurements and models using adaptive-beam antennas for outdoor urban cellular communications. IEEE Trans Antennas Propag, 61, 1850-1859(2013).
[39] LB Huang, X Li, WT Wan et al. Adaptive FDA radar transmit power allocation for target detection enhancement in clutter environment. IEEE Trans Veh Technol, 72, 11111-11121(2023).
[40] HL Wang, HF Ma, M Chen et al. A reconfigurable multifunctional metasurface for full‐space control of electromagnetic waves. Adv Funct Mater, 31, 2100275(2021).
[41] J Wang, J Kühne, T Karamanos et al. All‐dielectric crescent metasurface sensor driven by bound states in the continuum. Adv Funct Mater, 31, 2104652(2021).
[42] XY Wu, HY Feng, F Wan et al. An Ultrathin, Fast-Response, Large-Scale Liquid-Crystal-Facilitated Multi-Functional Reconfigurable Metasurface for Comprehensive Wavefront Modulation. Adv Mater, 36, 2402170(2024).
[43] TH Liu, WH Li, YY Meng et al. Six‐mode orbital angular momentum generator enabled by helicity‐assisted full‐space metasurface with flexible manipulation of phase, polarization, and spatial information. Adv Opt Mater, 10, 2102638(2022).
[44] Renzo M Di, A Zappone, M Debbah et al. Smart radio environments empowered by reconfigurable intelligent surfaces: how it works, state of research, and the road ahead. IEEE J Sel Areas Commun, 38, 2450-2525(2020).
[45] QQ Wu, R Zhang. Intelligent reflecting surface enhanced wireless network via joint active and passive beamforming. IEEE Trans Wirel Commun, 18, 5394-5409(2019).
[46] TJ Cui, MQ Qi, X Wan et al. Coding metamaterials, digital metamaterials and programmable metamaterials. Light Sci Appl, 3, e218(2014).
[47] RC Zhu, JF Wang, TS Qiu et al. Direct field-to-pattern monolithic design of holographic metasurface via residual encoder-decoder convolutional neural network. Opto-Electron Adv, 6, 220148(2023).
[48] C Qian, B Zheng, YC Shen et al. Deep-learning-enabled self-adaptive microwave cloak without human intervention. Nat Photonics, 14, 383-390(2020).
[49] Q Ma, GD Bai, HB Jing et al. Smart metasurface with self-adaptively reprogrammable functions. Light Sci Appl, 8, 98(2019).
[50] ZJ Luo, XY Ren, L Zhou et al. A high‐performance nonlinear metasurface for spatial‐wave absorption. Adv Funct Mater, 32, 2109544(2022).
[51] X Wan, JW Wang, ZA Huang et al. Space–time–frequency modulation mechanisms of monochromatic and nonmonochromatic electromagnetic waves on a digital programmable transmission metasurface. Adv Funct Mater, 32, 2107557(2022).
[52] M Ouyang, FF Gao, YC Wang et al. Computer vision-aided reconfigurable intelligent surface-based beam tracking: prototyping and experimental results. IEEE Trans Wirel Commun, 22, 8681-8693(2023).
[53] WH Li, Q Ma, C Liu et al. Intelligent metasurface system for automatic tracking of moving targets and wireless communications based on computer vision. Nat Commun, 14, 989(2023).
[54] B Wang, JH Liu, SJ Zhu et al. A dual-input moving object detection method in remote sensing image sequences via temporal semantics. Remote Sens, 15, 2230(2023).
[55] N Nigam, DP Singh, J Choudhary. A review of different components of the intelligent traffic management system (ITMS). Symmetry, 15, 583(2023).
[56] D Sha, JQ Gao, D Yang et al. Calibrating stochastic traffic simulation models for safety and operational measures based on vehicle conflict distributions obtained from aerial and traffic camera videos. Accid Anal Prev, 179, 106878(2023).
[57] GS Kong, HF Ma, BG Cai et al. Continuous leaky-wave scanning using periodically modulated spoof plasmonic waveguide. Sci Rep, 6, 29600(2016).
[58] Z Wang, SQ Li, XQ Zhang et al. Excite spoof surface plasmons with tailored wavefronts using high‐efficiency terahertz metasurfaces. Adv Sci, 7, 2000982(2020).
[59] A Bansal, CJ Panagamuwa, WG Whittow. Millimeter-wave beam steerable slot array antenna using an inter-digitated capacitor based corrugated SIW. IEEE Trans Antennas Propag, 70, 11761-11770(2022).
[60] Z Wang, Y Dong. Amplitude and frequency modulated leaky wave antenna for reconfigurable intelligent radiation. IEEE Trans Antennas Propag, 72, 2189-2201(2024).
[61] D Yang, S Nam. Frequency reconfigurable beam scanning squarely modulated reactance surface antenna with period and surface reactance control. IEEE Access, 11, 72552-72561(2023).
[62] SZ Wang, Z Li, B Wei et al. A Ka-band circularly polarized fixed-frequency beam-scanning leaky-wave antenna based on groove gap waveguide with consistent high gains. IEEE Trans Antennas Propag, 69, 1959-1969(2021).
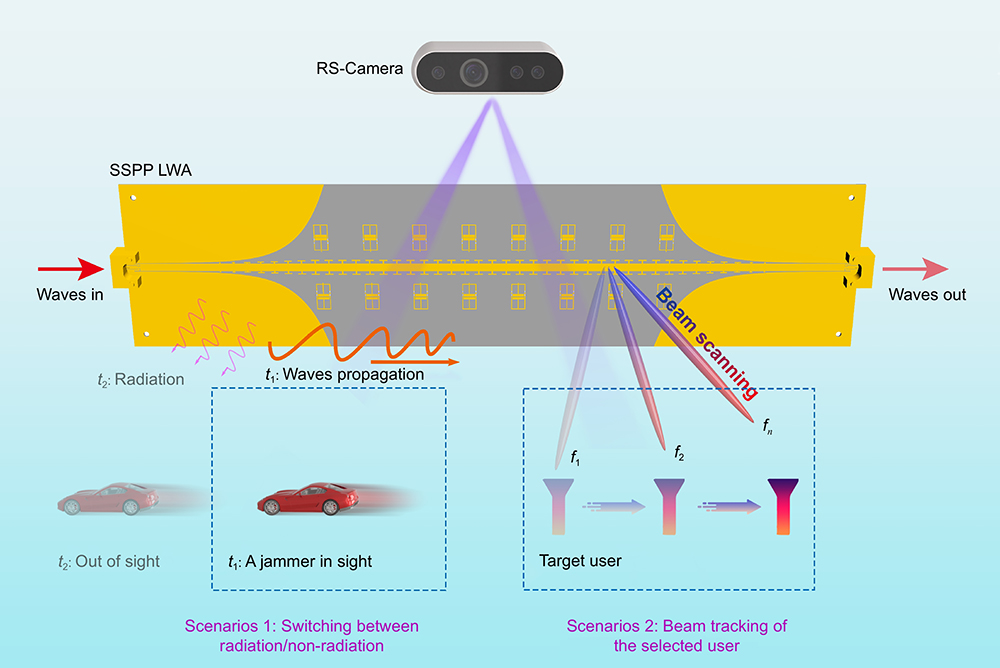
Set citation alerts for the article
Please enter your email address