Shaoshao Yu, Wenyu Zhao, Xin Wang, Xinghua Li, Shougang Zhang, "Novel compensation technique for mitigating dispersion in fiber-optic microwave frequency transfer systems," Chin. Opt. Lett. 23, 020601 (2025)

Search by keywords or author
- Chinese Optics Letters
- Vol. 23, Issue 2, 020601 (2025)
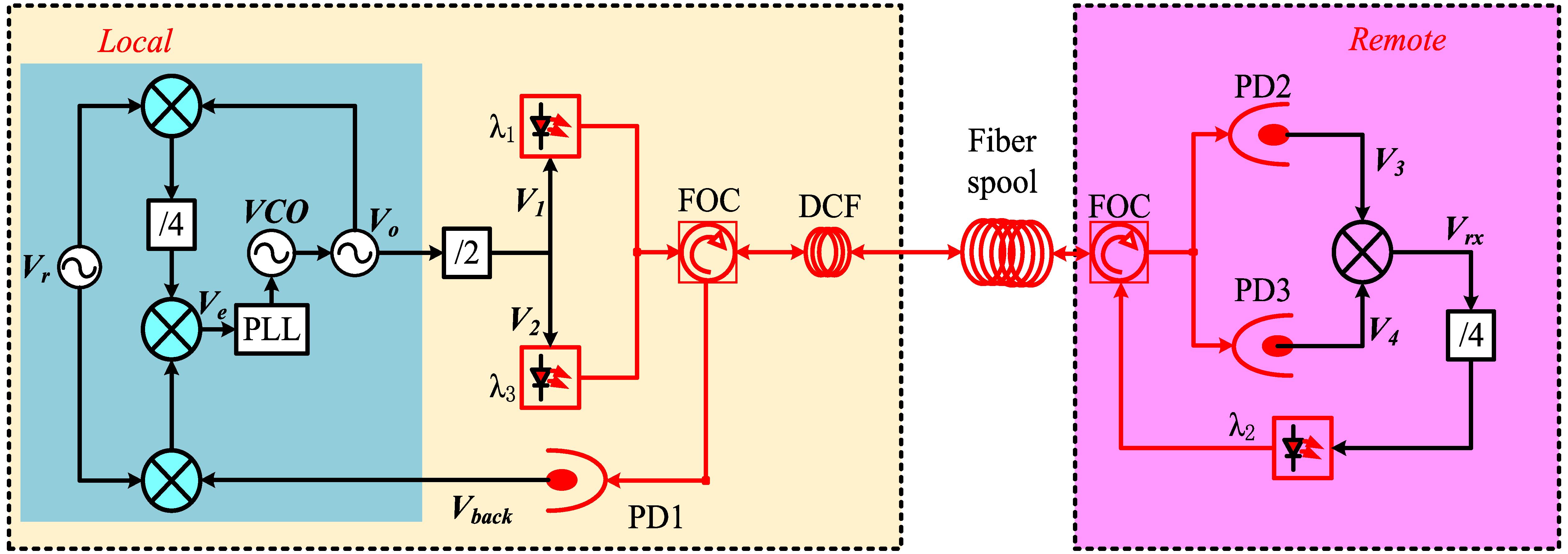
Fig. 1. Architecture of the compensation system for fiber-based microwave transfer. VCO, voltage-controlled oscillator; PLL, phase-locked loop; FOC, fiber optic circulator; DCF, dispersion-compensating fiber; PD, photodiode.

Fig. 2. Schematic diagram of the microwave frequency transfer system. OCXO, over-controlled crystal oscillator; PDRO, phase-locked dielectric resonant oscillator; MZM, Mach–Zehnder modulator; PS, polarization scrambler; WDM, wavelength division multiplexing; DCF, dispersion-compensating fiber; Bi-EDFA, bidirectional erbium-doped fiber amplifier.

Fig. 3. Temporal behaviors of the propagation delay of the previous scheme (black trace) and the propagation delay of the new scheme (red trace).

Fig. 4. Fractional frequency instability of the 100 km link: the previous scheme (black squares), the new scheme (red circles), and the system noise floor with an optical attenuation to shorten the link (blue triangles).

Fig. 5. Propagation delay variation as a function of the ambient temperature of the fiber link. (a) The previous scheme used laser wavelengths of 1550.92 and 1551.72 nm. (b) The previous scheme used laser wavelengths of 1551.72 and 1552.52 nm. (c) The new scheme used laser wavelengths of 1550.92, 1551.72, and 1552.52 nm.
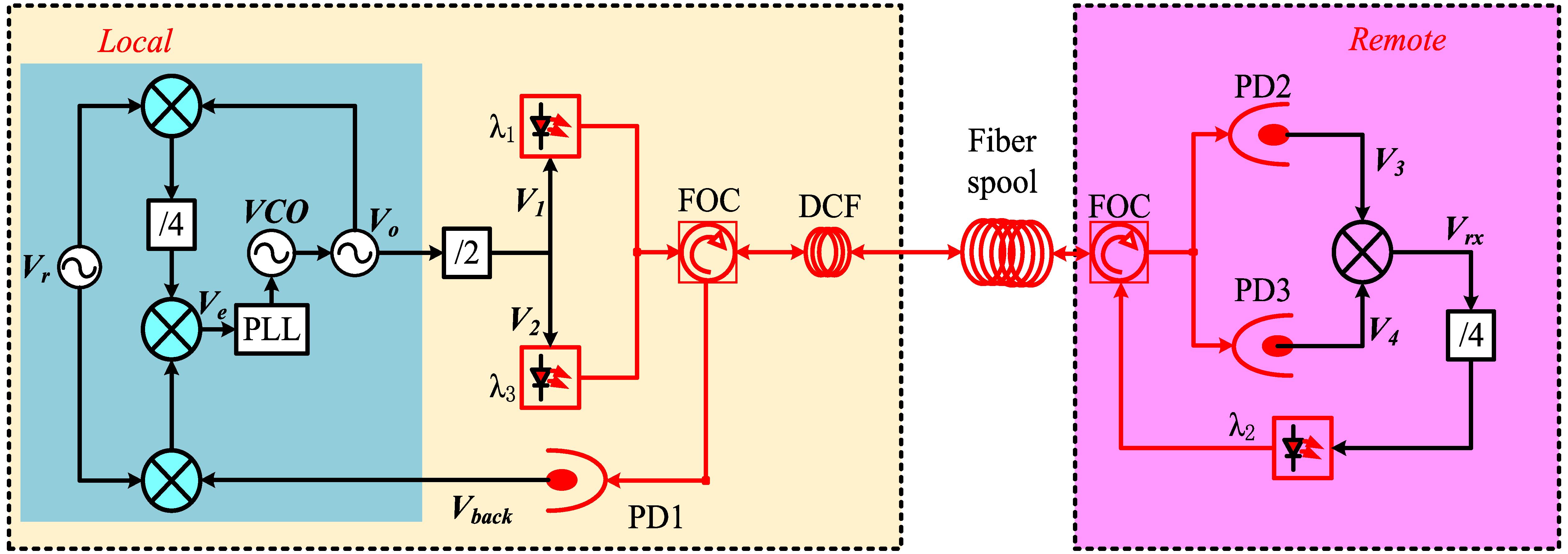
Set citation alerts for the article
Please enter your email address