[59] T.B. Toh, J.J. Lim, E.K. Chow, Epigenetics in cancer stem cells. Mol. Cancer 16, 29 (2017).

Search by keywords or author
- Nano-Micro Letters
- Vol. 16, Issue 1, 188 (2024)
References
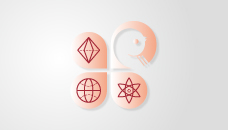
Afsana Sheikh, Prashant Kesharwani, Waleed H. Almalki, Salem Salman Almujri, Linxin Dai, Zhe-Sheng Chen, Amirhossein Sahebkar, Fei Gao. Understanding the Novel Approach of Nanoferroptosis for Cancer Therapy[J]. Nano-Micro Letters, 2024, 16(1): 188
Download Citation
Set citation alerts for the article
Please enter your email address