Peiming Li, Xiaojin Chen, Xiaodong Qiu, Binglin Chen, Lixiang Chen, Baoqing Sun, "Ghost imaging, development, and recent advances [Invited]," Chin. Opt. Lett. 22, 112701 (2024)

Search by keywords or author
- Chinese Optics Letters
- Vol. 22, Issue 11, 112701 (2024)
![(a) Experimental diagram of ghost imaging[1]. (b) Experimental diagram of ghost diffraction and interference[2].](/richHtml/col/2024/22/11/112701/img_001.jpg)
Fig. 1. (a) Experimental diagram of ghost imaging[1]. (b) Experimental diagram of ghost diffraction and interference[2].
![Experimental setup diagram of ghost imaging with a classical angle correlated source[6].](/richHtml/col/2024/22/11/112701/img_002.jpg)
Fig. 2. Experimental setup diagram of ghost imaging with a classical angle correlated source[6].
![Experimental setup diagram of ghost imaging with pseudo-thermal light source[12,13].](/Images/icon/loading.gif)
![Experimental setup of phase and amplitude retrieval in ghost diffraction from field-correlation measurements[26].](/Images/icon/loading.gif)
Fig. 4. Experimental setup of phase and amplitude retrieval in ghost diffraction from field-correlation measurements[26].
![Experimental setup for a CCD-based interferometer recording a hologram under spatially incoherent illumination[27].](/Images/icon/loading.gif)
Fig. 5. Experimental setup for a CCD-based interferometer recording a hologram under spatially incoherent illumination[27].
![Recording a hologram of a single-photon (HSP) with quantum interference[33]. (a) Sketch of the basic idea of HSP. (b) Two non-destructive quantum interference cases generating coincidence measurements.](/Images/icon/loading.gif)
Fig. 6. Recording a hologram of a single-photon (HSP) with quantum interference[33]. (a) Sketch of the basic idea of HSP. (b) Two non-destructive quantum interference cases generating coincidence measurements.
![Fourier ptychographic (FP) reconstruction of a portion in a USAF resolution test chart. (a) Image acquired with normal illumination. (b) Incoherent sum of the 225 images acquired for the acquisition. (c) Intensity reconstruction of the USAF target through FP technique. (d) Phase reconstruction of the USAF target through FP technique[31].](/Images/icon/loading.gif)
Fig. 7. Fourier ptychographic (FP) reconstruction of a portion in a USAF resolution test chart. (a) Image acquired with normal illumination. (b) Incoherent sum of the 225 images acquired for the acquisition. (c) Intensity reconstruction of the USAF target through FP technique. (d) Phase reconstruction of the USAF target through FP technique[31].
![Schematic of polarization entanglement-enabled quantum holography[32]. (a) Sketch of the experimental setup. (b) Phase image displayed on the Alice SLM. (c) Intensity image measured by the Alice EMCCD. (d) Intensity image measured by the Bob EMCCD. (e) The pattern displayed on the Bob SLM compensates for the static phase distortion. (f)–(i) Intensity correlation images measured by Bob for (f) 0, (g) π/2, (h) π, (i) 3π/2 phase shifts programmed on Bob SLM. (j) Reconstructed phase image.](/Images/icon/loading.gif)
Fig. 8. Schematic of polarization entanglement-enabled quantum holography[32]. (a) Sketch of the experimental setup. (b) Phase image displayed on the Alice SLM. (c) Intensity image measured by the Alice EMCCD. (d) Intensity image measured by the Bob EMCCD. (e) The pattern displayed on the Bob SLM compensates for the static phase distortion. (f)–(i) Intensity correlation images measured by Bob for (f) 0, (g) π/2, (h) π, (i) 3π/2 phase shifts programmed on Bob SLM. (j) Reconstructed phase image.
![Experimental setup and the state reconstruction of interferometric imaging of amplitude and phase of spatial biphoton states[61]. (a) Sketch of the experiment setup. (b) Reconstruct the phase-matching function by direct imaging. (c) Experimental correlations in the x- and y-coordinates obtained by placing the sensors in the image plane of the crystal. (d) Reconstructed phase and amplitude of a biphoton state.](/Images/icon/loading.gif)
Fig. 9. Experimental setup and the state reconstruction of interferometric imaging of amplitude and phase of spatial biphoton states[61]. (a) Sketch of the experiment setup. (b) Reconstruct the phase-matching function by direct imaging. (c) Experimental correlations in the x- and y-coordinates obtained by placing the sensors in the image plane of the crystal. (d) Reconstructed phase and amplitude of a biphoton state.
![Schematic diagram of quantum-enhanced wide-field phase imaging[63]. (a) Sketch of the experimental setup. (b) Detecting the birefringent phase samples. (c) Detecting the non-birefringent phase samples.](/Images/icon/loading.gif)
Fig. 10. Schematic diagram of quantum-enhanced wide-field phase imaging[63]. (a) Sketch of the experimental setup. (b) Detecting the birefringent phase samples. (c) Detecting the non-birefringent phase samples.
![Experimental setup of ghost imaging with the ICCD camera[34].](/Images/icon/loading.gif)
Fig. 11. Experimental setup of ghost imaging with the ICCD camera[34].
![Reconstructed images of a wasp’s wing[15]. (a) Image of the wing using 40,419 photons, (b) corresponding reconstructed image with λ = 5, (c) image of the wing using 738,298 photons, and (d) corresponding reconstructed image with λ = 10.](/Images/icon/loading.gif)
Fig. 12. Reconstructed images of a wasp’s wing[15]. (a) Image of the wing using 40,419 photons, (b) corresponding reconstructed image with λ = 5, (c) image of the wing using 738,298 photons, and (d) corresponding reconstructed image with λ = 10.
![Experimental setup for quantum imaging with interaction-free light[38].](/Images/icon/loading.gif)
Fig. 13. Experimental setup for quantum imaging with interaction-free light[38].
![Experimental setup for structured-pump-enabled quantum pattern recognition[42].](/Images/icon/loading.gif)
Fig. 14. Experimental setup for structured-pump-enabled quantum pattern recognition[42].
![Experimental results for human face recognition[42].](/Images/icon/loading.gif)
Fig. 15. Experimental results for human face recognition[42].
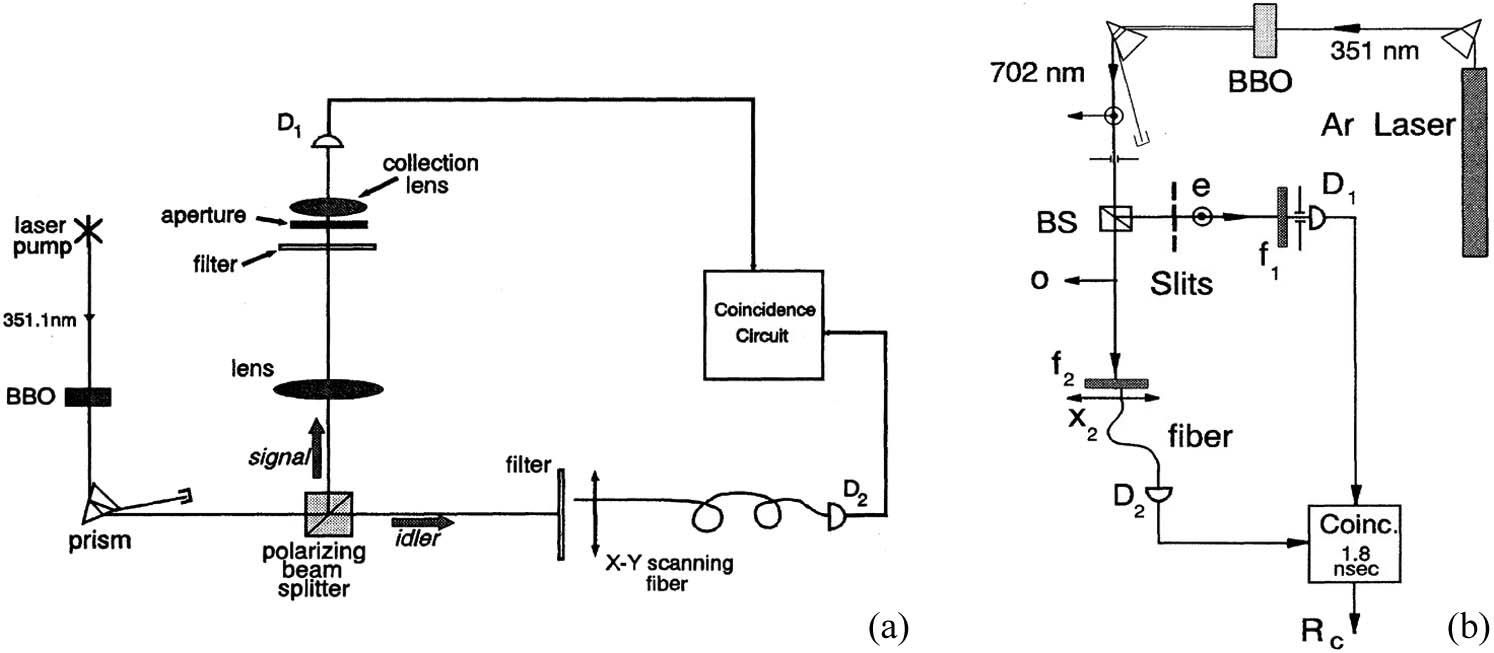
Set citation alerts for the article
Please enter your email address