Hongxing Dong, Linqi Chen, Xinjie Li, Zhanpeng Wang, Jingzhou Li, Zeyi Wang, Xuting Chen. Progress of Superfluorescence in Perovskite Quantum Dot Superlattices (Invited)[J]. Acta Optica Sinica (Online), 2025, 2(5): 0502001

Search by keywords or author
- Acta Optica Sinica (Online)
- Vol. 2, Issue 5, 0502001 (2025)
![Basic structure and properties of perovskite quantum dots. (a) Crystal structure of quantum dots of cesium lead halide perovskite[30]; (b) high-resolution transmission electron microscopic image of perovskite quantum dots[7]; (c) defect tolerance characteristics of perovskite quantum dots[32]; (d) fluorescence spectrum characteristics of perovskite quantum dots[7]; (e) absorption spectra and fluorescence lifetime of perovskite quantum dots[7]](/richHtml/aos_ol/2025/2/5/0502001/img_01.jpg)
Fig. 1. Basic structure and properties of perovskite quantum dots. (a) Crystal structure of quantum dots of cesium lead halide perovskite[30]; (b) high-resolution transmission electron microscopic image of perovskite quantum dots[7]; (c) defect tolerance characteristics of perovskite quantum dots[32]; (d) fluorescence spectrum characteristics of perovskite quantum dots[7]; (e) absorption spectra and fluorescence lifetime of perovskite quantum dots[7]
![Synthesis method of perovskite quantum dots. (a) Schematic diagram of the synthesis of perovskite quantum dots by hot injection method; (b) effect of ligand chain length on the synthesis of perovskite quantum dots by hot injection method[49]; (c) reaction equilibrium control of perovskite quantum dot synthesis[50]; (d) synthesis of perovskite quantum dots with oleic acid and oleylamine solvent[51]; (e) hot injection method synthesis of perovskite quantum dots with benzoyl halide as a halogen precursor[53]](/richHtml/aos_ol/2025/2/5/0502001/img_02.jpg)
Fig. 2. Synthesis method of perovskite quantum dots. (a) Schematic diagram of the synthesis of perovskite quantum dots by hot injection method; (b) effect of ligand chain length on the synthesis of perovskite quantum dots by hot injection method[49]; (c) reaction equilibrium control of perovskite quantum dot synthesis[50]; (d) synthesis of perovskite quantum dots with oleic acid and oleylamine solvent[51]; (e) hot injection method synthesis of perovskite quantum dots with benzoyl halide as a halogen precursor[53]
![Surface passivation of perovskite quantum dots. (a) Schematic diagram of defects in quantum dots[66]; (b) types of ligands on the surface of perovskite quantum dots[30]; (c) sodium ion passivation of perovskite quantum dot surface[83]; (d) alumina coating passivation of perovskite quantum dots[84]; (e) ligand exchange and SiO2 coating passivation of perovskite quantum dots[85]](/Images/icon/loading.gif)
Fig. 3. Surface passivation of perovskite quantum dots. (a) Schematic diagram of defects in quantum dots[66]; (b) types of ligands on the surface of perovskite quantum dots[30]; (c) sodium ion passivation of perovskite quantum dot surface[83]; (d) alumina coating passivation of perovskite quantum dots[84]; (e) ligand exchange and SiO2 coating passivation of perovskite quantum dots[85]
![Self-assembly method of quantum dots. (a) Schematic diagram of superlattice preparation by saturated solvent evaporation[14]; (b) device diagram of a saturated solvent evaporation method87]; (c) schematic diagram of perovskite quantum dot assembly by ultrasonic method[94]; (d) template method to control the self-assembly position and size of perovskite quantum dot superlattice[95]; (e) multicomponent superlattice films prepared at the liquid-liquid interface[96]](/Images/icon/loading.gif)
Fig. 4. Self-assembly method of quantum dots. (a) Schematic diagram of superlattice preparation by saturated solvent evaporation[14]; (b) device diagram of a saturated solvent evaporation method87]; (c) schematic diagram of perovskite quantum dot assembly by ultrasonic method[94]; (d) template method to control the self-assembly position and size of perovskite quantum dot superlattice[95]; (e) multicomponent superlattice films prepared at the liquid-liquid interface[96]
![Structural characterization methods for perovskite quantum dot superlattices. (a) TEM image of a CsPbBr3 quantum dot superlattices[102]; (b) HAADF-STEM image of CsPbBr3 quantum dot superlattices[14]; (c) schematic diagram of the working principle of GIWAXS and GISAXS[103]; (d) OA/OLA capped CsPbBr3 superlattice (left) and zwitterion-capped CsPbBr3 superlattice (right) with their GISAXS mapping and simulated crystallographic information[106]; (e) two-dimensional GISAXS pattern (on Si3N4 membrane)[88]; (f) GIWAXS patterns for CsPbBr3 and CsPbI3 films with different ligands[107]; (g) steady-state PL and absorption spectra of colloidal solutions of NPLs used to form superlattices with different face orientations and their corresponding 2D GIWAXS patterns (qz: out-of-plane scattering vector; qr: in-plane scattering vector)[108]](/Images/icon/loading.gif)
Fig. 5. Structural characterization methods for perovskite quantum dot superlattices. (a) TEM image of a CsPbBr3 quantum dot superlattices[102]; (b) HAADF-STEM image of CsPbBr3 quantum dot superlattices[14]; (c) schematic diagram of the working principle of GIWAXS and GISAXS[103]; (d) OA/OLA capped CsPbBr3 superlattice (left) and zwitterion-capped CsPbBr3 superlattice (right) with their GISAXS mapping and simulated crystallographic information[106]; (e) two-dimensional GISAXS pattern (on Si3N4 membrane)[88]; (f) GIWAXS patterns for CsPbBr3 and CsPbI3 films with different ligands[107]; (g) steady-state PL and absorption spectra of colloidal solutions of NPLs used to form superlattices with different face orientations and their corresponding 2D GIWAXS patterns (qz: out-of-plane scattering vector; qr: in-plane scattering vector)[108]
![Optical characteristics of superfluorescence (SF) in metal-halide perovskite quantum dot superlattices. (a) Basic processes and characteristics of SF[109]; (b) schematic diagram of the formation of SF in perovskite quantum dot superlattices[14]; (c) PL spectra of quantum dots and coupled quantum dot superlattices (left) and time-resolved PL decay of quantum dots and coupled quantum dot superlattices (right; blue: uncoupled quantum dots; red: coupled quantum dot superlattices)[14]; (d) time-resolved emission intensity trace under different excitation powers (left) and SF decay τSF (top right), SF emission intensity (middle right), and collective delay time τD (bottom right) as functions of laser power[14]; (e) HAADF-STEM images and SF emission spectrum of binary ABO3-type superlattices[88]; (f) schematic diagram of exciton coherence in perovskite quantum dot solids[113]](/Images/icon/loading.gif)
Fig. 6. Optical characteristics of superfluorescence (SF) in metal-halide perovskite quantum dot superlattices. (a) Basic processes and characteristics of SF[109]; (b) schematic diagram of the formation of SF in perovskite quantum dot superlattices[14]; (c) PL spectra of quantum dots and coupled quantum dot superlattices (left) and time-resolved PL decay of quantum dots and coupled quantum dot superlattices (right; blue: uncoupled quantum dots; red: coupled quantum dot superlattices)[14]; (d) time-resolved emission intensity trace under different excitation powers (left) and SF decay (top right), SF emission intensity (middle right), and collective delay time (bottom right) as functions of laser power[14]; (e) HAADF-STEM images and SF emission spectrum of binary ABO3-type superlattices[88]; (f) schematic diagram of exciton coherence in perovskite quantum dot solids[113]
![Exciton phase transitions, cavity-enhanced superfluorescence (CESF) characteristics, and their relationship with pump density in quantum dot superlattice microcavities. (a) Phase transitions of exciton ensembles during the self-assembly of quantum dots into superlattice microcavities (“+” and “-” represent holes and electrons, respectively)[114]; (b) time-resolved PL decay of SF and CESF[15]; (c) peak intensity Imax of SF/CESF signals vs pumping density[15]; (d) power dependences of radiative time tr[15]; (e)(f) schematic illustrations of the remaining dipoles after conventional laser and CESF processes[15]](/Images/icon/loading.gif)
Fig. 7. Exciton phase transitions, cavity-enhanced superfluorescence (CESF) characteristics, and their relationship with pump density in quantum dot superlattice microcavities. (a) Phase transitions of exciton ensembles during the self-assembly of quantum dots into superlattice microcavities (“+” and “-” represent holes and electrons, respectively)[114]; (b) time-resolved PL decay of SF and CESF[15]; (c) peak intensity Imax of SF/CESF signals vs pumping density[15]; (d) power dependences of radiative time tr[15]; (e)(f) schematic illustrations of the remaining dipoles after conventional laser and CESF processes[15]
![Development and application of CESF. (a) Experimental demonstration of quantum containers with ultrafast transition of exciton quantum coherence based on CsPbBr3 superlattice microcavities[15]; (b) nanosecond laser performance diagram of CsPbBr3 quantum dot superlattices [left: PL intensity and full width at half maximum (FWHM) vs pumping intensity; right: laser spectrum][83]; (c) time dynamics analysis of multi-mode CESF under different pumping densities[118]; (d) emission spectrum and fitted laser spectrum of typical CsPbBr2Cl superlattices as functions of power, and the inset shows two-dimensional optical WGM distribution[119]; (e) schematic diagram of low-threshold CESF process by preparing spherical quantum dot superlattices[120]](/Images/icon/loading.gif)
Fig. 8. Development and application of CESF. (a) Experimental demonstration of quantum containers with ultrafast transition of exciton quantum coherence based on CsPbBr3 superlattice microcavities[15]; (b) nanosecond laser performance diagram of CsPbBr3 quantum dot superlattices [left: PL intensity and full width at half maximum (FWHM) vs pumping intensity; right: laser spectrum][83]; (c) time dynamics analysis of multi-mode CESF under different pumping densities[118]; (d) emission spectrum and fitted laser spectrum of typical CsPbBr2Cl superlattices as functions of power, and the inset shows two-dimensional optical WGM distribution[119]; (e) schematic diagram of low-threshold CESF process by preparing spherical quantum dot superlattices[120]
![Novel physical mechanisms in CESF. (a) Physical pictures of the excited states and different radiation effects in QD and superlattice samples[24]; (b) echo-like SF with radiative energy fluctuations in a superlattice post-excitation pulse disturbance[24]; (c) physical explanation of the echo-like radiation [red-purple spheres: excitons; brown arrows passing through the spheres: cooperative radiation phase; blue halo: laser field introduced by the disturbance; orange-green background: virtual light field shared by cooperative (thermal) excitons; grid lines: unit quantum dots in the superlattice sample] [24]; (d) time-resolved PL image under different pump densities[114]; (e) schematic diagram of sample structure for generating cooperative exciton polaritons[25]; (f) angle-resolved PL spectra of a DBR-superlattice thin film excited above twice the pump threshold power[25]](/Images/icon/loading.gif)
Fig. 9. Novel physical mechanisms in CESF. (a) Physical pictures of the excited states and different radiation effects in QD and superlattice samples[24]; (b) echo-like SF with radiative energy fluctuations in a superlattice post-excitation pulse disturbance[24]; (c) physical explanation of the echo-like radiation [red-purple spheres: excitons; brown arrows passing through the spheres: cooperative radiation phase; blue halo: laser field introduced by the disturbance; orange-green background: virtual light field shared by cooperative (thermal) excitons; grid lines: unit quantum dots in the superlattice sample] [24]; (d) time-resolved PL image under different pump densities[114]; (e) schematic diagram of sample structure for generating cooperative exciton polaritons[25]; (f) angle-resolved PL spectra of a DBR-superlattice thin film excited above twice the pump threshold power[25]
![Application prospect of superfluorescence and cavity enhanced superfluorescence. (a) Second-order correlation measurement of superradiation in waveguide[128]; (b) schematic diagram of storage protocol for superradiant quantum memory[129]; (c) quantum optical properties of superradiant light sources[130]; (d) schematic diagram of experimental apparatus for free electron triggered superfluorescence[131]](/Images/icon/loading.gif)
Fig. 10. Application prospect of superfluorescence and cavity enhanced superfluorescence. (a) Second-order correlation measurement of superradiation in waveguide[128]; (b) schematic diagram of storage protocol for superradiant quantum memory[129]; (c) quantum optical properties of superradiant light sources[130]; (d) schematic diagram of experimental apparatus for free electron triggered superfluorescence[131]
|
Table 1. State-of-the-art superfluorescence and cavity-enhanced superfluorescence observations in perovskite quantum dot superlattces with key information
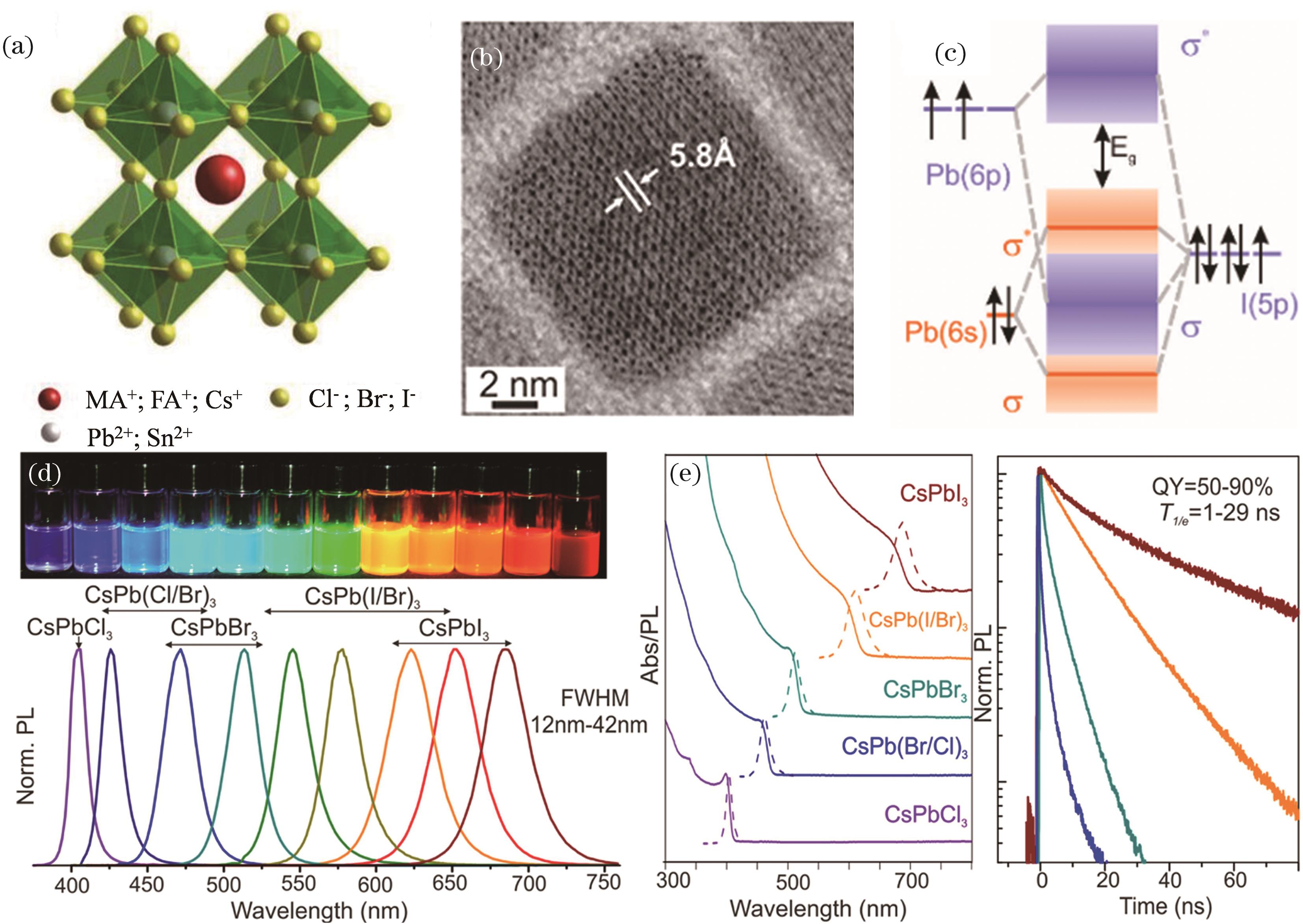
Set citation alerts for the article
Please enter your email address