Long Huang, Linhan Tang, Yang Wang, Minhui Cheng, B. E. Little, Sai T. Chu, Wei Zhao, Weiqiang Wang, Wenfu Zhang, "Multi-channel Hong–Ou–Mandle interference between independent comb-based weak coherent pulses," Photonics Res. 13, 837 (2025)

Search by keywords or author
- Photonics Research
- Vol. 13, Issue 4, 837 (2025)

Fig. 1. Schematic diagram of the principle of WCPs HOM interference. (a) Diagram of the principle of WCPs HOM interference; WCPs in paths a and b meet at a 50:50 BS and undergo HOM interference. (b) Typical HOM interference “dip”, with the right side (i)–(iii) indicating the relationship between photon indistinguishability and interference visibility at different optical delays.
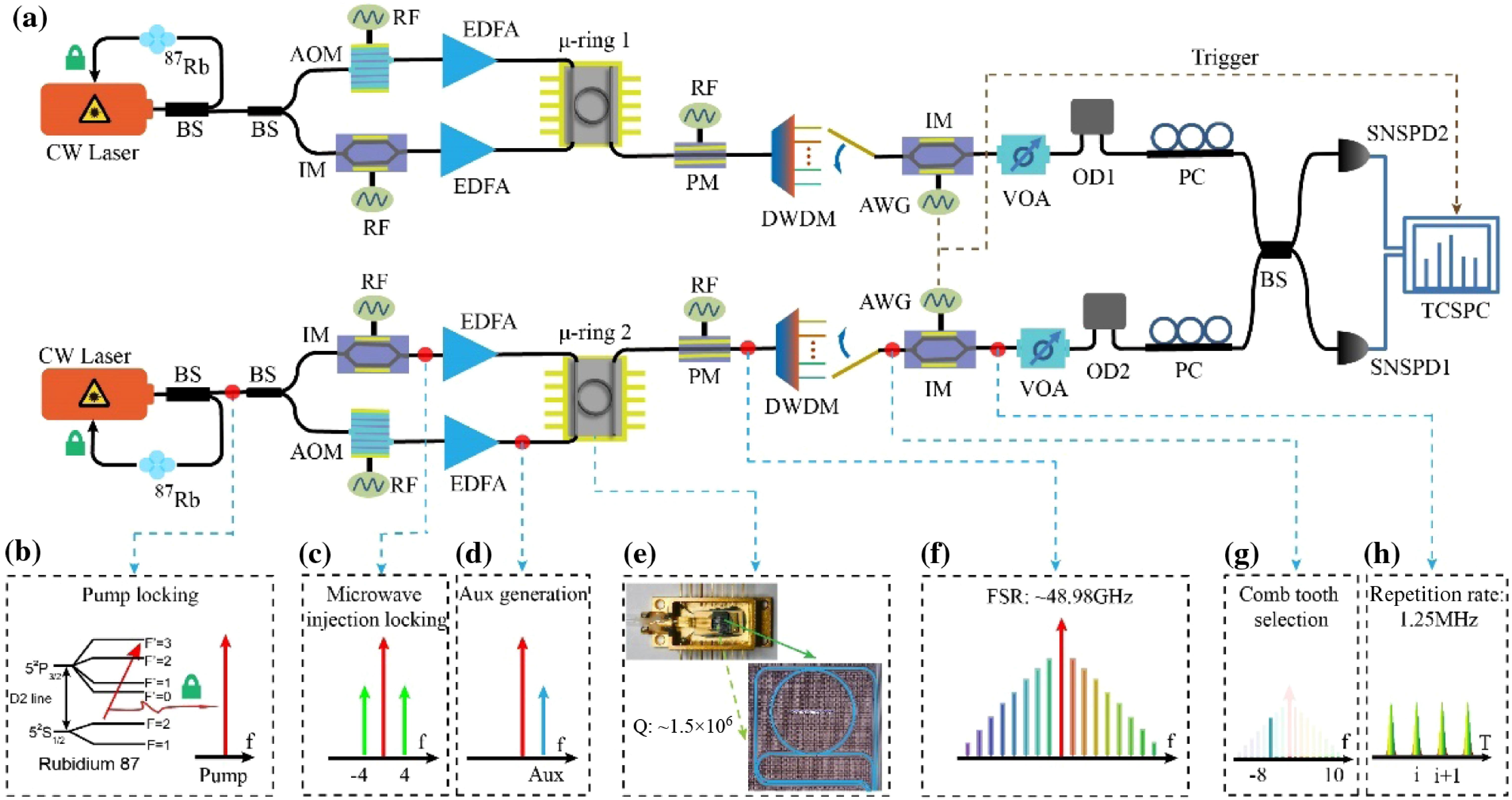
Fig. 2. Experimental setup. (a) Schematic diagram of the experimental principle of multi-path WCPs HOM interference based on independently generated SMCs. CW laser: continuous wave laser; R 87 b : rubidium atom; AOM: acousto-optic modulator; IM: intensity modulator; RF: radio frequency; EDFA: erbium-doped fiber amplifier; μ -ring: microring; PM: phase modulator; DWDM: dense wavelength division multiplexing; VOA: variable optical attenuator; OD: optical delay; PC: polarization controller; SNSPD: superconducting nanowire single-photon detector; TCSPC: time-correlated single-photon counting. (b) The pump laser frequency is locked to R 87 b atomic transition from F = 2 to F ′ = 3 . (c), (d) and (f)–(h) Spectral output from different optical elements. (e) The picture shows the 14-pin butterfly-packaged MRR. Inset: magnified view of the MRR observed under a microscope. The loaded quality (Q ) factor is ∼ 2.1 × 10 6 .
![Results of two independently fully locked SMCs. (a) Single-soliton state spectra of two independently generated SMCs. The top left inset shows the comb teeth selected in the experiment for generating WCPs, along with their numbering. (b) The recorded beat signals of two atomic transition referenced pump lasers. The two locked lasers have the same average frequency of 192.1140573 THz with a peak-peak frequency fluctuation of 0.247 MHz in an hour. (c) Repetition rate signals of two independently generated SMCs in the single-soliton state: unlocked [left, (c)] and locked [right, (c)]. When the repetition rates are locked to ultra-stable microwave signals, the jitter of the repetition rate signal is reduced by three orders of magnitude compared to the unlocked state.](/Images/icon/loading.gif)
Fig. 3. Results of two independently fully locked SMCs. (a) Single-soliton state spectra of two independently generated SMCs. The top left inset shows the comb teeth selected in the experiment for generating WCPs, along with their numbering. (b) The recorded beat signals of two atomic transition referenced pump lasers. The two locked lasers have the same average frequency of 192.1140573 THz with a peak-peak frequency fluctuation of 0.247 MHz in an hour. (c) Repetition rate signals of two independently generated SMCs in the single-soliton state: unlocked [left, (c)] and locked [right, (c)]. When the repetition rates are locked to ultra-stable microwave signals, the jitter of the repetition rate signal is reduced by three orders of magnitude compared to the unlocked state.

Fig. 4. WCPs generation. (a) The comb tooth of the SMC is modulated into pulses with an FWHM of ∼ 200 ps using an IM. Inset: actual pulse fitted with theoretical Gaussian pulse. (b) The number of single photons from two-path independently generated WCPs recorded by the SNSPDs before interference. When no light is incident on the single-photon detector, the measured dark count rate of the single-photon counter is ∼ 80 counts per second.

Fig. 5. HOM interference between two independently generated WCPs based on the SMCs. (a) The normalized coincidence counts between SNSPD1 and SNSPD2 versus the temporal misalignment adjusted by OD1 and OD2. The 16 channels in (a) correspond to the selected comb teeth shown in the inset of Fig. 2 (a). (b) HOM interference fringe of channel 3 between two independent WCPs. The fringe visibility is estimated to be 46.69 % ± 0.85 % . (c) The HOM interference fringe visibility of 16 pairs of independent WCPs. The visibility is distributed in the range of 46.02%–47.17% with maximum standard deviation of ± 0.94 % for five measurements.

Fig. 6. Packaging of the MRR and generation of SMC. (a) Experimental setup diagram for the generation of fully locked SMC. Dashed box: schematic diagram of the modulation transfer spectroscopy method for locking the pump laser frequency. (b) Loaded Q -factor of the Hydex material micro-ring resonator used in the experiment. (c) Butterfly package of the MRR (c-I) and microscope photograph (c-II).

Set citation alerts for the article
Please enter your email address